. Introduction
A considerable share of tangible cultural heritage consists of ruins and buildings. Some of them are monumental and of historical interest, some of them of local interest or remaining to be discovered. Often such objects lack a chronologic frame, and the method that would provide a time for buildings is not a straightforward radiometric dating. For millennia mortar was the prevailing one among other materials used in constructions until the early 1st half of the 20th century. Following the industrial revolution and expansion of new technologies, the traditional mortar has been replaced by cement. This industrial product, although developed using experience gained over millennia, is rather useless for radiocarbon dating as it contains old carbon additions, resulting in ages as high as tens of thousands of years.
Mortars, except for hydraulic, pozzolana and cocciopesto (Ringbom et al., 2011), can be more suitable for radiocarbon dating. The mechanism of binding CO2 from the atmosphere shown by the slacked carbonate oxide (carbonate hydroxide) is a perfect analog to the photosynthetic path of building carbon into organic matter. Early on, radiocarbon researchers tried to apply this method to dating archeological and historical monuments. The first results were encouraging (Labeyrie and Delibrias, 1964) but followed by less successful attempts (Stuiver et al., 1965). The main challenge in the preparation of mortars is the separation of old carbon, which might be included in the binder due to incomplete burning. Contamination can also be added together with aggregates such as sand and gravel. Although other complications of 14C signal in mortars can occur, such as delayed hardening, fire damage, or formation of new carbonates, the old carbon is the prevailing problem. Therefore, the first attempt is to achieve the most reliable 14C ages focused on the removal of geological carbonate. The observed difference between the reactivity of the binder, which dissolves faster, and the limestone has been used to separate the contaminant.
A ‘revolution’ has only been brought about by the application of the AMS method (Nelson et al., 1977).
The minimal quantities of carbon needed for the AMS 14C analyses open an opportunity for measurements of multiple dissolution fractions. Developed independently by two teams (Heinemeier et al., 1997), the method has been modified and adopted by few laboratories. Also, a little different approach has been proposed by Nawrocka et al. (2005) and Marzaioli et al. (2011). As well, this method underwent various modifications and, at some stage, has been combined with sequential dissolution (Michalska and Czernik, 2015).
The reliability of the radiocarbon ages of mortar has been debated ever since the first disappointing results of Stuiver et al. (1965) have been published. A considerable effort has been made by the mortar 14C dating community to establish the procedure and protocols as well as quality control (Ringbom et al., 2014). The results of MODIS inter-comparison exercise (Hajdas et al., 2017 and Hayen et al., 2017) have shown that some of the mortars cannot be dated by 14C method and that the understanding of mortar geochemical characteristics is a key to understating these problematic results (Michalska et al., 2017). Here, we present results of radiocarbon dating of a monument, which has been dated only by typology.
. Site and material
The site of Hohenrätien (GR) is located on a rock rising 250 m above the Viamala Valley (Fig. 1a) overseeing the roads of the San Bernardino and the Splügen Pass, which connect the Swiss Valley of Hinterreihn with the Italian Valle San Giacomo and Chiavenna (Fig. 1b).
The transit from Northern Italy to the Rhine Valleys appreciated since the Bronze and the Iron Age, was also used by the Romans. In the medieval ages, the strategic location was chosen for the construction of the castle, which in years 1996−1997 was a subject of archeological prospections and investigations. Moreover, in 1999, the owner of the castle discovered additional remains of older construction. The archeological excavations 2001−2004 documented an early Christian church (Gairhos and Janosa, 2011). The latter phases of constructions of the whole monument could be dated by dendrochronology, and a wiggle-matching of 14C dated tree rings to 1180−1210 AD (Gairhos et al., 2005). However, the earlier phases could only be dated by typology; therefore, the radiocarbon dating of mortar is one possibility to provide a numeric date on the monument.
The location of the three mortar samples collected from the remains of construction A (Bau A) is shown in Fig. 2.
Fig 2
Location of the mortar samples analyzed in this study (Figure modified from (Gairhos and Janosa 2011)).
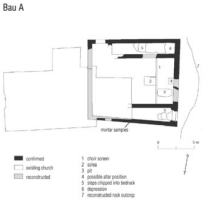
. Methods
Preparation of mortar for radiocarbon dating followed the protocol developed so far at ETH Laboratory (Hajdas et al., 2017 and Hajdas et al., 2020). The principle of the method is a separation of suitable grain size and discrimination between anthropogenic and geogenic carbonate by a different dissolution time. Two samples (Nos. 891 and 894) were prepared using the method of sequential dissolution, which targets the fast-dissolving component of the binder. In the case of sample No. 891, two different grain size fractions: 45−63 μm and 32−63 μm were analyzed1; in the case of sample No. 894, only fraction of 45−63 μm was used. For sequential dissolution, sub-samples containing ca. 50 mg of powder were placed in one of the chambers of the special dual-chamber-glass vessel. The second chamber was filled with 10 ml of concentrated phosphoric acid (85% H3PO4). The vessel was then closed and evacuated at room temperature before pouring of acid to the chamber, which contained mortar. This process was timed, and freezing of purified (passing through a water trap) CO2 in LN was performed in sequence: four consecutive fractions were collected after each 3-second interval. The carbon content of each collected fraction was measured, and 10−100 μg of C was trapped in a 4-mm tube to be flame-sealed for analysis using Gas Ion Source (GIS) AMS facility at ETHZ (Ruff et al., 2010). The third sample of mortar (No. 897) contained visible lime lump (LL), which was used without sieving (the bulk of LL). This sample was sufficiently large; therefore, it was dissolved and graphitized to be measured using the MICADAS at ETH Zurich (Synal et al., 2007). Solid- and gas-formed samples were analyzed together with the corresponding size of standard (Oxa2) and background samples (C-1, IAEA).
. Results and discussion
The outcome of the radiocarbon dating performed on samples from the Hohenrätien old parish church is summarized in Table 1. With the exception of one sample, radiocarbon ages of the fast fractions (1st and 2nd, i.e. 1−3 s and 4−6 s) show close 14C ages (at 2-sigma error level) for all three preparations. Slow fractions (3rd and 4th, i.e. >7 s dissolution time) are older, which shows the presence of the old (geological) component. The ages obtained on samples <100 μg using GIS have higher uncertainly than the one measurement on the lime lump, which was graphitized. The lime lump shows age, which is in agreement with the ages of the 1st fast fraction. However, due to the high uncertainty of the GIS measurements, the ages of the sequential dissolution cannot help to evaluate if the 14C age of lime lump is older than the selected 1st fraction. The three ages of the 1st fraction from the three independent preparation can be combined to 1495±41 BP (X2-Test: df=2 T=5.3 (5% 6.0)) and all the 1st fraction ages can also be combined with the age of the lime lump. The resulting age is 1551±21 BP (X2-Test: df=3 T=7.7 (5% 7.8)). Calendar ages of mortar samples were obtained for radiocarbon ages, which are considered accurate (Table 1). OxCal v4.3.2 online calibration software was used (Ramsey, 2017) with the INTCAL13 calibration data set (Reimer et al., 2013).
Table 1
Results of the 14C AMS analysis of mortar samples. All the samples but lime lump (LL) were analyzed using GIS. Combined and calibrated ages were obtained using OxCal v4.3.2 (only for 14C ages evaluated as accurate).
Lab Code | Sample/Fraction (μm) | Dissolution time (s) | 14C age ±1 sigma (BP) | Calibrated age (95.4% conf. level) (AD) | μg C |
---|---|---|---|---|---|
ETH-65530 | 891, 45−63 | 1−3 | 1534±84 | 348−656 AD | 95 |
ETH-65530 | 891, 45−63 | 4−6 | 1688±88 | NA | 109 |
ETH-65530 | 891, 45−63 | 7−9 | 2108±102 | NA | 108 |
ETH-65530 | 891, 45−63 | 10−12 | 2621±101 | NA | 104 |
ETH-65530 | 891, 32−45 | 1−3 | 1605±74 | 257−606 AD | 60 |
ETH-65530 | 891, 32−45 | 4−6 | 1737±83 | NA | 105 |
ETH-65530 | 891, 32−45 | 7−9 | 2144±103 | NA | 88 |
ETH-65530 | 891, 32−45 | 10−12 | 2461±105 | NA | 96 |
ETH-69913 | 894, 45−63 | 1−3 | 1386±64 | 543−770 AD | 81 |
ETH-69913 | 894, 45−63 | 4−6 | 1581±63 | NA | 99 |
ETH-69913 | 894, 45−63 | 7−9 | 1664±77 | NA | 91 |
ETH-69913 | 894, 45−63 | 10−12 | 2254±76 | NA | 82 |
ETH-85506 | 897, LL (lime lumps) | Total dissolution | 1569±24 | 422−547 AD | 1200* |
Combined | 891 & 894 | all 1−3 s | 1495±41 | 430−646 AD | X2-Test: df=2 T=5.3 (5% 6.0) |
Combined | 891 & 894 & 897 | all 1−3 s & LL | 1551±21 | 427−559 AD | X2-Test: df=3 T=7.7 (5% 7.8) |
Figure 3 shows all the results of the radiocarbon dating of all the samples and their evaluation. Following the procedure outlined in Hajdas et al., (2020), the radiocarbon ages of the fast-dissolving fractions: 1st: 1−3 s and 2nd: 4−6 s, have the potential of providing the accurate 14C signal for the time of binding the mortar. The slight increase in the ages of the 3rd and 4th fractions indicates the presence of the old, geological component. To establish a laboratory procedure for calculating the following is applied: only the 1st, i.e. the fastest fraction, is considered if the following fractions are not coherent. In an ideal case, if the following 2nd fractions of all three samples were in close agreement with the 1st fraction, a weighted mean can be calculated. Here, however, such combination failed the X2-Test; therefore, only 1st fractions were combined. In addition to the radiocarbon ages of the separated fast fractions of samples Nos. 891 and 894, a sample of lime lump from No. 897 was also analyzed as a whole, which showed a radiocarbon age that is in agreement with the 1st fractions of the three preparations. The calibration curve for the early medieval times 400−800 AD has a complicated nature. Moreover, the uncertainty of 14C ages obtained using GIS is higher. As a result, the calibrated ranges of the three samples were wide (Table 1, Figs. 4 and 5). The youngest of the radiocarbon ages dates the mortar to the period between 543 and 770 AD (Fig. 4). The combined calibrated age (weighted mean) of the mortar sample from the Hohenrätien church dates the mortar to 427−559 AD (Fig. 5). The typological dating of this monument points to the 5th/6th century AD (Gairhos and Janosa 2011), indicating a broad agreement of the obtained 14C chronology of mortar.
Fig 3
Radiocarbon ages of the three samples obtained after sequential dissolution in 3-second intervals. The first fraction was the collection of CO2 after the first 3 seconds and the consecutive fractions were collected in 3-second intervals (x-axis shows dissolution fractions: 1=1st fraction 1−3 s; 2= 2nd fraction 4−6 s; 3= 3rd fraction 7−9 s; 4=4th fraction 10−12 s). The red square marks the age of the lime lump.
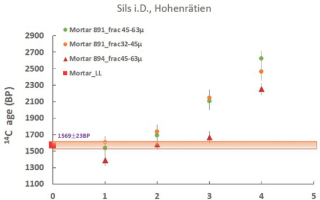
. Conclusions
Radiocarbon dating of the mortar provides the potential to date archeological and historic buildings. The early church at the Hohenrätien is an excellent example of the potential for the numeric dating method to be applied to mortar. The resulting radiocarbon ages of the three samples date the monument to the period between 257 and 770 AD. The wide range of calendar ages is due to the nature of the calibration curve and the age plateau between 420 and 530 AD. Nevertheless, the combined age of the fast component of the mortar and a single lime lump results in an age of 427−559 AD, confirming the typological dating. In summary, this study adds information about the reliability in using the 1st, i.e. the fastest dissolution fraction. Given the complexity of mortars, building a collection of well-dated sites with consistent mortar ages based on 1st fraction should be the goal of mortar dating projects.