. Introduction
Worldwide, agriculture is not only being affected by global warming and climate change but also accelerated soil erosion and the associated soil degradation (Walling, 1998; Marestoni et al., 2009). Accelerated soil erosion is one of the main environmental problem (Zapata and Agudo, 2000; Konz et al., 2010; Othman and Ismail, 2012) that largely affects sustainable use of soil and water resources (Patrocinio and Andrello, 2009). Soil erosion is a major threat to soil productivity and represents the main mechanism of land degradation worldwide (IAEA, 2014). According to ICOLD (2009), 27% of land in Africa is degraded, out of which 65% of agricultural land is degraded due to erosion and/or chemical and physical damage. In addition, soil erosion is reported by Collins et al. (2001) as a serious environmental problem in many African countries. The severe soil erosion and degradation largely affect food security in Sub-Saharan Africa (Dercon et al., 2012). This has been attributed to many developing countries in Africa having experienced expansion of agriculture on to marginal land. To tackle the loss of productive soil, quantification of soil erosion rates is a major requirement (Poręba, 2006; Porto et al., 2014b). Further, soil erosion and deposition have offsite effects related to loss of reservoir storage capacity. In water resources, most reservoirs do not fulfil their functions due to capacity loss and reduction in water quality resulting from sedimentation (Marestoni et al., 2009; Apostu et al., 2012).
Globally, the loss of reservoir storage capacity has been approximated to 0.5–1% per year (ICOLD, 2009). This greatly affects the reservoir functions like water supply for domestic, irrigation and hydropower generation. On the other hand, water quality is lowered especially when the source of sediments is from agricultural farms or contaminated environment. The eroded sediment acts as a vector for the transportation of agrochemicals from agricultural lands to water bodies (Porto and Walling, 2012). Further, due to climate change and global warming, water erosion is expected to be on the increase (Mabit et al., 2014c). An increase in extreme daily rainfall has been experienced in many catchments and this may lead to severe loss of soil in cultivated land. As a result, there is need to estimate the chronology of sediment production especially in most catchments within developing countries where the sedimentation rates of reservoirs are inadequately documented. Also, soil erosion rate data at different temporal changes is required so as to aid in quantifying potential changes that might occur when different land uses have been adopted and with change in climate (Li et al., 2010; Benmansour et al., 2013).
To reconstruct the history on impacts of anthropogenic activities in water bodies, sediment collected from lakes play a major role. The collected sediments also aids in establishing accurate chronologies of sedimentation and in study of sediment accumulation rates (IAEA, 2003). The use of radionuclides in soil erosion and deposition studies allows assessment of soil erosion and deposition within a catchment to be obtained with minimal field visits (IAEA, 1998; Walling, 1999; Saç and İçhedef, 2015). The Fallout radionuclides (FRNs) is a cost-effective tool that is useful in studying soil redistribution due to erosion within the landscape from plot to basin scale. Also, the information derived from FRNs is useful in complementing information provided by conventional erosion measurements and modelling (IAEA, 2014).
The traditional methods of quantifying soil erosion rate are labour intensive and require long term monitoring programs (Poręba, 2006). Walling and Quine (1992), reported that existing soil erosion monitoring techniques has many limitations in terms of their potential to provide information on longer term soil loss rates over large areas. In addition, in most developing countries especially in Africa, continuous sediment gauging has not been done consistently for longer periods. To tackle these challenges, FRNs have been adopted in many developed countries to assess soil erosion magnitudes (Zapata and Agudo, 2000; Dercon et al., 2012; Porto and Walling, 2012) but little has been done in Africa. Therefore, there is need to explore the use of FRNs in assessing soil erosion rates and patterns of soil redistribution/deposition. The theory of using isotopes in soil erosion analysis is based on their characteristics. The isotopes reach the soil through precipitation and they are then strongly bound to the soil particles where they can only be moved by erosion (Saç and İçhedef, 2015). The use of radionuclides in determining soil erosion rates, aids in modelling soil redistribution within the catchment or the landscape (Nouira et al., 2003; Porto et al., 2014b). Further, use of the radionuclides provides a fast and robust way of determining spatial soil redistribution (Meusburger et al., 2013). Use of FRNs, provides data that is useful in targeting the areas with high erosion and sediments transfers. The identified areas can then be targeted for land management and conservation practices thus reducing the negative impacts resulting from soil erosion. Successful use of FRNs require suitable and statistically sound sampling procedures (Mabit et al., 2013). This highly reduces the limitations associated with the use of FRNs in soil redistribution studies. Care and proper selection of reference sites is required for proper application of FRNs in assessing soil redistribution. In addition, there is need for more research in various parts of the world on use of FRNs and assessing their limitations as challenged by Parsons and Foster, 2011. This would lead to an improved and highly viable method of assessing erosion and deposition.
In Africa and most developing countries, very few studies have been conducted in using FRNs to evaluate erosion and deposition magnitudes. The aim of this paper is to provide information on application of FRNs in soil erosion, deposition and reservoir sedimentation studies in Africa.
. Fallout radionuclides (FRNs)
Some of the highly used radionuclides include; 137Cs, 210Pb and 7Be (Dercon et al., 2012; Porto et al., 2014a). In applying these nuclides, selection of a reference site is of critical importance. This aids in improving validity of the technique to accurately quantify erosion and deposition. To convert 137Cs, 210Pb and 7Be activity to soil erosion, deposition or distribution rate, several models have been developed (Walling et al., 2002; Walling et al., 2014) and they are summarised in Table 1. For successful application of the models, a sound understanding of the models and the meaning and derivation of their parameters is highly required to reduce the limitation and uncertainty of information obtained.
Table 1
Models used in Converting FRNs to Erosion /depositional rates.
Model | Input parameter | FRNs and site used | Advantage | Limitation |
---|---|---|---|---|
Proportional model | Plough depth, bulk density | 137Cs (Cultivated) | 1.Requires few input parameters 2.Easy to apply | 1.Oversimplification of 137Cs accumulation in the soil 2.Model does not account for dilution of 137Cs concentrations in the soil |
Mass Balance Model 1 (MBM1) | Plough depth, bulk density, year of tillage commencement proportional factor, relaxation depth, annual fallout flux* | 137Cs (Cultivated) | 1. Accounts for progressive reduction in the 137Cs concentration of the soil | 1.Model does not account for removal of freshly deposited 137Cs by erosion during a rainfall event 2.The model assumes that concentration of 137Cs in eroded sediments remains constant through time. Also, the concentration of deposited sediment is assumed to be constant |
Mass balance model 2 (MBM2) | Tillage depth, tillage constant, proportional factor, tillage relaxation depth, slope length and slope gradient for each section of the transect, annual fallout flux* | 137Cs (Cultivated) and 210Pb (Cultivated) | 1.Accounts for tillage effects 2.Caters for 137Cs dilution in the plough layer 3.Obtained results are more realistic than from the previous models | More data is required than from the previous models |
Mass Balance Model 3 (MBM3) | In addition to MB2 parameters Diffusion coefficient, relaxation depth, migration, coefficient, annual fallout flux* | 137Cs (Cultivated) and 210Pb (Cultivated) | 1.Results from this model are closer to reality 2.It accounts for tillage redistribution in flow direction | The model is data intensive |
Profile shape model | Profile shape factor, Mass depth of soil | 137Cs (Uncultivated) 7Be (Cultivated) | Simple and easy to use | Does not account for temporal variation of 137Cs |
Diffusion and migration and model | Diffusion coefficient, Migration rate, /retardation factor | 210Pb (Uncultivated) | 1. Accounts of temporal variation of depth profile resulting from the time-dependent fallout input | Requires more time and data to use |
The use of 137Cs in soil erosion and deposition studies
The 137Cs is an artificial radionuclide which primarily originated from nuclear weapons testing (Ritchie and Mchenry, 1990; Walling, 1998). It was first detected in early 1950s peaking in early 1960s (Stefano et al., 1999; Mabit et al., 2014a) before the nuclear test ban treaty. However, it declined to very low levels in the mid-1970s. The 137Cs isotope has a half-life of about 30 years (Poręba, 2006). Gellis et al. (2006), Walling (1998), Mahawatte and Abeynayake (2010) observed that there is a strong adsorption of 137Cs by clay and organic matter in soils. According to Patrocinio and Andrello (2009) and Dercon et al. (2012) 137Cs is useful in studying the sediment chronology since it is rapidly and strongly absorbed to fine particles of the soil. Also, the distribution of 137Cs in the soil sediment profile is directly related to the chronology of sediment deposition. Its fallout deposition on land surface from atmospheric nuclear test occurs from rainfall. It is largely absorbed on finer organic and soil particles and it resists leaching through the soil profile (Walling et al., 1995). The profiles of 137Cs in sediments give the deposition history that can be interpreted to assign calendar dates.
According to Mabit et al. (2014d) when assessing the redistribution rates of the soil using 137Cs, the measured values of 137Cs in individual sampling points are compared with reference 137Cs input. The reference inventory represents 137Cs values measured in undisturbed sites where no erosion nor deposition has taken place (Othman and Ismail, 2012). To reduce the variability of data collected hence ensure that there is uniform local distribution of 137Cs, reference sites should be selected in the vicinity of the targeted fields that will be subsequently investigated (Mabit et al., 2013). Selecting appropriate local reference inventory is therefore a major requirement to successful use FRNS in soil erosion and deposition assessment. If the 137Cs activity is lower than that of reference site, this signifies erosion. On the other hand, if the 137Cs activity value is higher than that of the reference site, it signifies that soil deposition has taken place (Othman and Ismail, 2012; Porto et al., 2014a). Mostly, in natural uncultivated soils, the distribution of 137Cs has an exponential decline with soil depth.
According to Walling and Quine (1992), a sharp decline in 137Cs activity is observed with increasing depth. Minimum vertical translocation of 137Cs was also reported. In different soils studied, more than 75% of the total inventory occurs in the top 15 cm.
The key assumption that affects application of 137Cs is that uniform spatial distribution of the nuclide exists (Parsons and Foster, 2011). Local variability of 137Cs is influenced by individual storm. The application assumes that the patterns from individual storms over extended period leads to uniform local pattern of total fallout (Walling and Quine, 1992).In addition, it is assumed that redistribution of 137Cs indicates that there is soil movement and that 137Cs is rapidly adsorbed by soil particles (Mabit et al., 2014a; Walling and Quine, 1992).
Despite these assumptions the use of FRNs provides more information on soil and deposition status in different sites. This method provides an immense potential in data scarce regions. To further improve on the findings the choice of reference sites is critical. Parsons and Foster (2011) reported that use of reference sites in assessing soil erosion and deposition limits the application of FRNs. They argued that samples collected from the same reference sites give high variability of 137Cs. Another limitation raised by Parsons and Foster (2011), on the use of 137Cs is its variability in areas affected by the Chernobyl accident, where additional radio-caesium fallout inputs in 1986 can complicate interpretation of 137Cs measurements. In such areas, use of 210Pb alone or in combination with 137Cs in assessing erosion and deposition rates would be highly encouraged.”
Although, possibilities of using 137Cs in assessing soil erosion and sedimentation in developing countries was discussed in 1995 (IAEA, 1998) very little has been done in most African countries. Since nuclear fallout was low in southern hemisphere regions, it has been noted that 137Cs, is on decline compared to northern hemisphere (Ritchie and Mchenry, 1990; Walling, 1998; Stefano et al., 1999; Dercon et al., 2012). A great part of sub-Saharan Africa falls on the southern hemisphere. Hence there is difficulty in using 137Cs, for soil erosion and deposition, since the quantities measured are very low or in some cases below detection limit. As a result, erosion rates can be assessed using a natural radionuclide such as 210Pb.
Use of 210Pb in Soil erosion and deposition
This is a natural radionuclide that originates from the decay of 222Rn, a stable isotope of Radon (Rn) that occurs in soil and the atmosphere. It is a natural radioactive form of lead found in small quantities in most soils as part of uranium decay series and has a half-life of 22.3 years (Lubis, 2006; Porto et al., 2006). According to Guzman et al. (2013) 210Pb is usually derived from radioactive decay of 222Rn (Radon 222) gas daughter of Radium 226 (226Ra). The 226Ra occurs naturally in soils and rocks and generates 210Pb that is in equilibrium with its parent material (Zhang et al., 2014). The soil and sediment samples for measuring 210Pb is stored for about a month before analysis so as to achieve equilibrium of 226Ra and its 222Rn (Shakhashiro and Mabit, 2011; Mabit et al., 2014c). The concentration of 210Pb is greatest at the surface in uncultivated land because of its continuous replenishment at the soil surface. Also, it has an exponential reduction in activity below the surface (Zhang et al., 2007). The nuclide is useful in establishing the chronology of sediment deposits and estimating the rate of accumulation of such deposits.
The rate of 210Pb activity declines with depth in a core and it aids in establishing an age depth relationship and the rate of sediment accumulation. In cases where accumulation rates are high, the 210Pb activity declines slowly with depth. On the other hand, if accumulation rates are low, the 210Pb activity reduces rapidly with depth (Walling et al., 2002). The 210Pb is useful in estimating the soil redistribution for up to 100 years (Zheng et al., 2006; Benmansour et al., 2014; Mabit et al., 2014a) a period longer than that of 137Cs. Further, 210Pb is useful in areas where low 137Cs concentration is likely to pose a problem especially in the southern hemisphere (Porto et al., 2006; Shakhashiro and Mabit, 2011).
A research conducted in different regions under Coordinated Research Project (CRP), aimed at investigating the potential of using 210Pb measurements to estimate soil redistribution rates. It reported that only 31% of the participants provided acceptable results for total 210Pb analyes (IAEA, 2011). These findings are further supported by Golosov et al. (2011) who reported that 210Pb was characterised by high local variability compared to 137Cs. Almost similar observation were reported by Mabit et al. (2014c) where a major limitation in use of 210Pb was reported to be the difficulty experienced in accurately analysing the nuclide using gamma spectrometry. There are seasonal variations of 210Pb in the atmosphere and this affects its use in some parts of the world. Therefore, there is need for more research to test and validate the use of 210Pbex for documenting soil redistribution rates under a range of different environmental and climatic conditions (Benmansour et al., 2014).
Although use of 210Pb and 137Cs in erosion assessment over longer periods is possible, their application for short time period is very limited. However, other radionuclides such as 7Be nuclide can be used.
7Be use in soil erosion and deposition studies
This is a naturally occurring cosmogenic radionuclide that is produced in the stratosphere and troposphere when oxygen and nitrogen spallate. The nuclide is then deposited as fallout (Guzman et al., 2013). According to Marestoni et al. (2009), 7Be is a radionuclide that is useful in assessing soil erosion over a short period and in identifying the newly deposited sediment. It is mostly detectable near the surface of the sediment samples and should be counted soon enough after a sample collection since it has a half-life of 53.3 days (Zapata and Agudo, 2000). According to Wilkinson et al. (2006), the nuclide is not found below 20 mm of any soil and the radionuclide is useful for surface sediments recovery. The concentration of 7Be in the soil is influenced by atmospheric processes, vertical transport in the troposphere and horizontal transport of subtropical and middle latitudes to the tropics and Polar Regions. In addition, it is used in studying mass exchange between troposphere and stratosphere, wet and dry deposition (Marestoni et al., 2009). The main fallout process of 7Be is through precipitation which takes 95% of the total. When 7Be is used in assessing sedimentation, the stable, eroding and aggrading sites are well distinguished using both their total inventory and the vertical profile distribution. An exponential decline in activity concentration and areal activity density with depth is usually experienced. Loss of 7Be from surface soil indicates that soil erosion has taken place and this removes the upper section of the exponential profile leading to a reduction in overall inventory (Mabit et al., 2014b). The 7Be nuclide are useful in assessing the effectiveness of recent soil erosion conservation practices. They are also used to document erosion and sedimentation rates associated with rainfall events. The application of 7Be has two key assumptions; the input of 7Be associated with the erosional or depositional event is spatially uniform, and, that any pre-existing 7Be is uniformly distributed across the area under investigation. Since the method is dependent on individual event, the 7Be inventories may be low in arid and semi-arid area of land. Though the nuclide has been used in soil erosion, redistribution and deposition analyses, its application in Africa is very limited.
. Application of fallout radionuclides in Africa
This section reviews application of FRNs in assessing soil erosion and reservoir sedimentation in Africa. In this, the continent was divided into four regions namely; Eastern Africa, Northern Africa, Western Africa and Southern Africa. During the review, one recent project was found to cover almost all the above-named regions i.e. were well distributed in the African region. The project has been implemented in 10 countries namely; Algeria, Benin, Cote d’ivore, Madagascar, Mali, Morocco, Senegal, Tunisia, Uganda and Zimbabwe. It focused on using Fallout radionuclide’s (137Cs, 210Pb and 7Be) in quantifying African soil degradation, soil erosion and sediment redistribution rates within agricultural landscapes. The results from this IAEA conducted research project had not yet been published at the period this review paper was compiled. In this paper, the summary of radionuclide activities within the reference site is presented in Table 2. In addition, Fig. 1 shows the distribution of the radionuclide use in Africa. The other findings for different application of FRNs in African region were presented as follows;
Fig. 1
Map of Africa showing Regions where FRNs have been used for soil erosion and Reservoir sedimentation analysis.
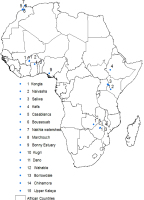
Table 2
Summary of radionuclide activity in reference sites.
Country (Region) | Author | Reference inventory (Bq/m2) | FRN used |
---|---|---|---|
Uganda (Kongta) | Ruecker et al., 2008 | 392 | 137Cs |
(Magada) | 439 | 137Cs | |
Kenya | Jeff and Shepherd, 2009 | <30 (Below minimum detectable limits) | 137Cs |
Ethiopia | Denboba, 2005 | 2064 ± 112 | 137Cs |
(Kefa zone) | |||
Morocco (Boussouab) | 1450 | 137Cs | |
Sadiki et al., 2007 | 3305 | 210Pb | |
Benmansour et al.,2013 | 1445 | 137Cs | |
101 | 7Be | ||
Bukina Faso (Dano and Wahable) | Schmengler, 2011 | 729 ± 14 | 137Cs |
Ghana | Pennock, 2000 | 925.1 | 137Cs |
Niger (Southwest) | Chappell et al., 1998 | 2066 ± 125 | 137Cs |
Zimbabwe | Quine et al., 1993 | 30 | 137Cs |
Zambia (Kaleya) | Walling et al., 2003 | 2602 | 210Pb |
(Collins et al, 2001) | 202.0 ± 22.8 | 137Cs |
Eastern Africa
In this region Uganda, Kenya and Ethiopia were found to have some information on use of FRNs in soil and sediment studies. For instance, Ruecker et al. (2008) examined the potential of using 137Cs technique to assess soil redistribution rates in humid tropics of Uganda. The 137Cs inventory was found to be 392 and 439 Bq/m2 for Kongta and Magada reference sites, respectively. These results were found to be within the range of globally interpolated 137Cs inventory of the region. The average 137Cs inventory within Kongta and Magada was reported to be 200 ± 100 and 400 ± 100 Bq/m2 respectively. Within the Kongta and Magada sites the mean 137Cs inventory was reported to be 202 ± 87.2 and 382 ± 155 Bq/m2 respectively. Further, the net soil redistribution of the two sites (Kongta and Magada) indicated that there was soil loss of 21 ± 12.2 t/ha/yr and 4.5 ± 2.3 t/ha/yr, respectively. Soil deposition within Kongta and Magada was also reported to be 5.8 ± 0.9 and 6.3 ± 4.3 t/ha/yr respectively. It was concluded that 137Cs method was applicable in estimating soil redistribution within humid tropics of Africa. The study found out that peak hillslope erosion for Kongta and Magada were 44.6 t/ha/yr and 36.3 t/ha/yr while sedimentation rates were 6.4 t/ha/yr and 25 t/ha/yr respectively. The reduced soil deposition and increased soil erosion in Kongta is because the site is on steep hillslope. It was also reported that there had been no documented application of this method in the humid tropics of Africa. The low application of this method could be due to the region being on the southern hemisphere where 137Cs inventories are almost one order of magnitude lower than in the northern hemisphere (Walling and He, 1999; Collins et al., 2001; Walling et al., 2002). Ruecker et al. (2008) reported difficulty in selecting reference sites near the study area since intensive land use in this climatic zone has been practiced leaving almost all areas being disturbed. They also pointed out that lack of access to institutions with gamma spectrometer detectors in this region further affected widespread applicability of the 137Cs method.
Further, in Lake Naivasha, Kenya, a sediment core sample was analysed by Stoof-Leichsenring et al. (2011) who reported that, total 210Pb activity reached equilibrium with 226Ra at a depth of about 33 cm. Also, the unsupported 210Pb was reported to reduce irregularly with depth but the concentration in the top 15 cm was relatively constant at a mean rate of 164 Bq/kg. Also, at a depth below 30 cm, the concentration was found to be close or near the minimum detection limit. On the other hand, the 137Cs concentration was found to be highest between depths 20–25 cm. Stoof–Leichsenring et al. (2011) also used 210Pb dating to establish the core chronology that provided information for a period of 186 yr history of natural and human induced environmental changes.
In addition, Jeff and Shepherd (2009) analysed 137Cs soil samples in Sauwa river watershed, western Kenya. Four reference sites were selected in the study area and they consisted of sloped grassed pasture, thickly vegetated (Trees and shrubs) and two sites within Kapolete National forest. The sample depth intervals were too broad hence detailed soil profile analysis could not be undertaken. The samples were analysed for 137Cs and 210Pb using standard laboratory procedures in IAEA standard reference material (IAEA – 300). The sample counts were taken for 21.7 to 26.1 hours depending on nuclide activity. They reported that most of the samples had low concentration of the nuclide which was well below minimum detection concentration limits of 30 Bq/kg. The 137Cs was reported to be more concentrated in the upper few centimetres of the soil profile and this concentration decreased with soil depth. Further, little 137Cs was detected below a soil depth of 20 cm. The mean 137Cs concentration was reported to be 1.68 and 4.15 Bq/kg in eroded and non-eroded sites. Within the top soils, 82% of samples had detectable level of 137Cs while 33% had detectable levels within the sub soils. They confirmed the results by field visits that were conducted and 63% of the top soil was found to have been eroded. Almost similar findings were reported for Ethiopia by Denboba (2005).
Denboba (2005) conducted research on the high mountain forests in Kefa zone in southwest of Ethiopia, on the cultivated fields of selected subcatchments. The 137Cs was used to estimate the rate of soil erosion and deposition. It was found that there was an exponential decline of 137Cs activity with increase in depth. Further, 69% of 137Cs inventory was found in the upper 10 cm of soil surface while 19% and 12% inventories were found in 20 cm and 30 cm subsurface layers, respectively. The mean reference inventory was found out to be larger than the assumed range of 200–500 Bq/m2 (Collins et al., 2001) for the equitorial region. This pointed out the need of more studies within the equitorial region so as to understand the 137Cs activity other than relying on the world interpolated 137Cs activity inventories.
Northern Africa
The 137Cs was used by Sadiki et al. (2007) to quantify erosion rates and identify main factors involved in erosion process in Boussouab catchment, Eastern Rif Morocco. It was found out that at the sampling sites, the 137Cs activity ranged between 245 and 3670 Bq/m2. This indicated that in all sampling sites, erosion had taken place. In addition, soil loss was reported to be highly variable within land use and the variance ranged between 5.1 and 48.8 t/ha/yr. Further, the average soil loss was reported to increase by more than 100% when slope increased from 10° to 25° with loss of 17.7 t/ha/yr and 40.8 t/ha/yr, respectively. The radiometric analysis proved useful in quantifying erosion rates and in examining the soil movement pattern.
This view is supported by Benmansour et al. (2013) in a study conducted in Marchouch South East, Morocco. The findings reported that the net soil erosion rates were 14 t/ha/yr and 12 t/ha/yr when 137Cs and 210Pb were respectively used in assessing the soil redistribution in agricultural fields. The study further highlighted that water erosion is the leading process of soil redistribution in cultivated fields. In addition, Benmansour et al. (2013) conducted a research on estimating soil redistribution rates and assessment of effectiveness on soil conservation techniques using 210Pb and 137Cs isotopes in Marchouch South East from Rabat, Morocco. The net soil erosion using 137Cs, 210Pb and conventional method were reported to be 14.3 t/ha/yr, 12.1 t/ha/yr and 12.3 t/ha/yr, respectively. During the analysis of 137Cs, Mass Balance Model (MBM2) and MBM3 conversion models were used. The models yielded net soil redistributions of 14.3 t/ha/yr and 15.5 t/ha/yr, respectively. Within the reference site, it was observed that 137Cs and 210Pb were mainly distributed in the first 10 cm of the soil depth where the highest concentration was close to the surface and it then diminished with increase in soil depth. They reported that gross erosion and deposition rates in the study area were 12.9 and 0.8 t/hr/yr respectively. The use of combined 137Cs and 210Pb FRNs indicated that a potential exists on the use of nuclides in estimating long term erosion rates in agricultural fields in Morocco. The overall data generated on soil redistribution from two nuclides was similar.
Although the concentration of 137Cs is comparatively low in West African environments, results clearly differentiate zones of sediment loss and gain. The redistribution map of a hillslope in southwestern Burkina Faso shows maximum erosion rates of 16 t ha–1 yr–1 at the summit and shoulder position of the hillslope and maximum deposition rates of more than 20 t ha–1 yr–1 at the foot slope/valley position (Benmansour et al., 2013)
Benmansour et al., 2014 (in IAEA) assessed long term soil redistribution in a small agricultural field in Morocco using both 137Cs and 210Pb. 45 samples were collected along five (5) parallel transects and their activity was measured. The activities were converted into soil redistribution rates using MBM2. They found that 210Pb inventories in the study field ranged between 1700 and 5000 Bq/m2. This had a mean relative uncertainty for 210Pb ranging from 4.6 to 6.6%. The erosion rate was reported to range between 8 and 27 t/ha/yr. Also, in Marchouch Morocco, the 7Be was reported by Benmansour et al. (2011) in IAEA, (2011) to be successful in quantifying erosion rates during individual or heavy rainfall events. The potential of using 7Be in assessing the effectiveness of soil conservation measures was also demonstrated in Morocco in a FAO/IAEA Conducted Research Project (Mabit et al., 2014d). The 7Be was found in the first few centimetres of top soil since it has a short halflife and it does not easily move deep down before it degrades. Thus, the findings of availability of these nuclides with depth indicate that during radiometric analysis of soil and sediment samples, the top part of soil/sediment should be carefully analysed. In addition, during sample analysis, shorter interval sectioning along the depth should be adopted.
Western Africa
Schmengler (2011) found out that two cultivated sites at Dano and Wahable in Burkina Faso had 137Cs areal activity of 200 to 1500 Bq/m2 and 100 to 2100 Bq/m2, respectively. In the two study areas, it was observed that the upper part of the hillslope experienced erosion leading to low 137Cs inventories while the lower part of the hillslope had higher 137Cs inventories indicating deposition. Schmengler (2011) reported that the middle part had a balance between erosion process and sediment deposition from the upslope. The net erosion rates for the hill slopes in Dano and Wahable ranged between 2.4–3.3 t/ha/yr and 2.7–5 t/ha/yr respectively. At Bonny estuary in Nigeria, Babatunde et al. (2015), studied the different concentration ranges of various radionuclides on sediments. It was found that 137Cs activity concentration, was lowest in all the study sites. The 137Cs ranged between 39–67 Bq/kg while 226Ra ranged between 193–484 Bq/kg. In addition, 238U, 232Th and 40K were analysed, the results showed a range between 383–812 Bq/kg, 370–819 Bq/kg and 300–9800 Bq/kg for each of the radionuclides, respectively. The elevated 238U, 232Th and 40K in the region, could be attributed to the presence of cement factories along the banks of the estuary. They reported that cement has high background values of the three nuclides which are associated with limestone, shale, clay, gypsum and other cement raw materials. They reported that the concentration of 238U, 232Th and 40K in sediment samples was higher than that in raw cement samples of Nigeria. The 137Cs and 226Ra radionuclides activity concentrations were not further discussed in the paper and no information exists to link the work to erosion and sedimentation rates. Though the results showed that these radionuclides exist in the sediment core there is need for more investigation to fully understand the results.
Further, measurable quantities of 137Cs were found in soil samples from profiles in Southwest Niger with a net soil flux of 16 ± 2 t/ha/yr (Chappell et al., 1998). On the other hand, in Kugri farm, Ghana, 137Cs activity was determined by counting 662 KeV gamma emmissions in a period of 2 to 21 hours counting interval. According to Pennock (2000), and (IAEA, 2014) it was reported that though analysis was run on different sized fraction of the concentrations, no measurable 137Cs was detected. This indicated that, in the region of the study, the 137Cs would not be applicable in study of soil erosion and deposition and hence other FRNs like 210Pb should be exploited.
Southern Africa
An assessment of soil erosion within agricultural land in two contrasting sites (Borrowdale and Chinamora) near Harare, Zimbabwe was conducted by Quine et al. (1993) using 137Cs. The 137Cs technique was reported to be applicable in studying soil erosion within the region. Borrowdle area was characterised by commercial farming and tropical red soils while Chinamora area had communal farming and granite sandy soils. Within the undisturbed regions of both study sites, the reference inventory was found to be 30 Bq/m2 while the profile distribution on cultivated soils showed a mixed 137Cs throughout the plough layer. In addition, applicability of 210Pb technique in assessing soil loss and deposition under different land uses was investigated by Collins et al. (2001) and Walling et al. (2003) in upper Kaleya River basin, Zambia.
Collins et al. (2001) used 137Cs for different land uses. The considered land uses included; commercial cultivation, communal cultivation and bush grazing. The sample count time of 50,000s was used due to low activity of nuclide. During the study, general guidelines and criteria by Walling and Quine (1993) for identifying representative reference sites were followed. 36 core samples were collected in sites with minimum slopes, fully vegetated and where no deposition and erosion has taken place. Sampling was stratified along the transect to cover areas below where communal cultivation, commercial cultivation and bush grazing land use were practiced. The theoretical MBM for 137Cs was used. The gross erosion, net soil loss and deposition within communal cultivated site reported to be 7.0, 2.5 and 4.5 t/ha/yr while that of commercial cultivation was 7.0, 4.3 and 2.7 t/ha/yr respectively. In bush grazing site, gross erosion was lowest at 3.4 t/ha/yr and net soil loss and deposition was reported to be 2.9 and 0.5 t/ha/yr. These results were based on transects sampled within areas characterised by a given land use and not on the entire topographic unit (slope to river channel)
Walling et al. (2003) compared 137Cs and 210Pb in estimating the net soil redistribution under different land uses in upper Kaleya River basin, Zambia. The results from commercial cultivation gave soil loss amounts of 4.3 t/ha/yr and 4.5 /ha/yr while those of communal cultivation were 2.5 t/ha/yr and 1.2 t/ha/yr, respectively. Further, bush grazing had a net soil redistribution of 2.9 t/ha/yr and 3.7 t/ha/yr respectively. In the study using 210Pb, soil deposition was found to be 3.7, 5.2 and 4.5 t/ha/yr in commercially, communal cultivated and bush grazing land uses respectively. The findings by Walling et al. (2003) are comparable with those of Collins et al. (2001) who used 137Cs within the same catchment. The 137Cs and 210Pb has reported similar trend in gross erosion, net soil loss and deposition assessment. Also, it was reported that unsupported 210Pb can be used as an alternative to 137Cs in equatorial region of Africa where 137Cs inventories are very low.
. Conclusion
A challenge still exists in selecting suitable reference sites for radionuclides. There is need for more actual measurements of erosion using different methods to improve on the quality of results. In addition, the reference sites should be selected as close as possible to the areas erosion and deposition is being assessed. Although use of FRNs have been reported to be useful in assessing soil redistribution and reservoir sedimentation in different regions of world, there is a long way to go in applying FRNs in Africa. Hence more research is required to exploit the use of FRNs for soil erosion and reservoir sedimentation analysis. Some of the FRNs that have been used include; 137Cs, 210Pb and 7Be. Most of these radionuclide activities in Africa have been found detectable up to a maximum depth of 30 cm. Morocco in Northern Africa is one of the country in which a lot has been done from 2000s’ in the use of FRNs in soil erosion and soil redistribution assessment. In the southern Africa, high 137Cs activity was reported back in 1993. This radionuclide activity could have diminished with time hence the need for a more current study.
In the summarised review on African continent, most studies that have been conducted, are on use of 137Cs fallout radionuclide. In some cases, comparative study has been undertaken using 137Cs and 210Pb. The results from the reference inventory measured, indicated that 210Pb activity is higher than the 137Cs activity. Also in some regions, the 137Cs activity was reported to be below the minimum detection limit implying that the technique cannot be used in assessing soil erosion and deposition and that more studies/research are required for the region. Further, the highest 137Cs activity in the reference site has been reported in Niger at a value of 2066 ± 125 Bq/m2 a region that is in the northern hemisphere which received more of 137Cs activity than southern hemisphere.
Though the application of 137Cs in the southern hemisphere is minimal, due to its low 137Cs activity, the use of other fallout radionuclides such as 210Pb and 7Be need to be explored. As a result, more studies are required in Africa on application of 210Pb in areas that would be representative of the whole continent to better understand soil erosion processes and reservoir sedimentation. Also, 7Be nuclide is least applied within Africa. Thus, to understand short term effects on soil loss/redistribution, 7Be should be analysed. To improve on assessing soil loss, redistribution and deposition analysis in Africa, FRNs should be highly exploited. From the reviewed literature on Africa use of FRNs, only in Lake Naivasha Kenya, have they been applied to study sedimentation and sediment chronology. This indicates that there is need to link use of FRNs in the erosion and deposition studies within the catchment to sedimentation of reservoirs. This can be achieved by analysing more sediment cores from reservoirs to generate sedimentation rates and chronology.