. Introduction
Chronology is a crucial scientific question for both archaeologic and paleoenvironmental studies on the Qinghai-Tibetan Plateau (QTP). However, archaeological studies in the Qaidam Basin (QB), NE QTP, are hindered. In the QB, very few early archaeological sites were found, because of the erosional environment, which is not suitable for the preservation of human activity remains (HARs). As a result, artifacts were usually found on the ground surface, e.g., Xiao Qaidam site (Huang et al., 1987) and Lenghu site (Owen et al., 2006, Brantingham and Gao, 2006), and their ages could be only estimated by dating the underlying sediments, which might be much older than the human activities (Sun et al., 2010; Brantingham and Gao, 2006). Fortunately, aeolian sediments under depositional environment in the eastern QB preserve HARs well and offer opportunities to get robust chronology by both Optically Stimulated Luminescence (OSL) and 14C dating.
Methodologically, both OSL dating and 14C dating should be suitable for archaeological sites in aeolian sediments. Hou et al. (2012) dated Erfang archaeological site in the Guanting Basin (Fig. 1A), northeastern edge of the QTP, and the OSL (2.6 ± 0.2 ka) and AMS 14C (2401 ± 6 Cal BP) ages are in agreement within error. Sun et al. (2012) dated a hearth from the Jiangxigou archaeological site in the Qinghai Lake Basin (Fig. 1B), and offered four OSL ages around the hearth ranging from 12.9 ± 0.9 to 14.4 ± 1.0 ka, confirming the corresponding AMS 14C ages of 14690 ± 150 and 14760 ± 150 Cal BP (Madsen et al., 2006). Zeng (2006) dated two cultural layers (3229 ± 82 and 3477 ± 63 Cal BP after calibration) covered by aeolian sand in Nuomuhong town (Fig. 1B), southern QB, indicating severe aridity since ca. 3.3 ka. Though without direct OSL ages comparisons, this change matched the desertification and climate changes in other parts of the QB based on OSL dating (Yu and Lai, 2012, 2014; Zhou et al., 2012; Yu et al., 2013). These studies showed agreement between OSL and 14C dating and offered reliable chronologies for these sites; however, more disagreements were found with the increasing amount of ages.
Fig. 1
Geomorphology of the study region and location of the sections. The yellow dots with red centers show the archaeological sites mentioned in this studies, e.g., Yutian site (Han et al., 2014), Erfang site (Hou et al., 2012), Xiao Qaidam site (Huang et al., 1987, Brantingham and Gao, 2006; Sun et al., 2010), Lenghu site in the northern QB (Owen et al., 2006, Brantingham and Gao, 2006), Nuomuhong site (Zeng, 2006), Jiangxigou site (e.g., Madsen et al., 2006; Brantingham and Gao, 2006; Sun et al., 2012), and some sites in this study (HSZ, HBC and XRH). The red dots with white centers display the towns and sections in this region, respectively.
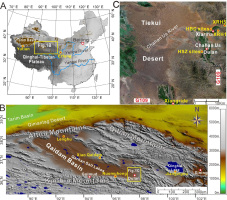
In the Yutian site (Fig. 1A), northwestern margin of the QTP, a hearth was dated by two OSL (7.72 ± 0.41 ka and 7.98 ± 0.47 ka) and four 14C ages (7564–7279, 7253–7006, 7409–7006, and 7276–6942 Cal BP), though comparable within errors, the OSL ages were systemically older than the 14C ages (Fig. 1A, Han et al., 2014). Finally the age of this site were proposed to be ca. 7.0–7.6 ka based mainly on 14C ages. In the eastern QB, Niu et al. (2010) reported six OSL and four 14C ages from aeolian sections containing HARs and found age disagreements from two layers. They obtained two OSL (1.14 ± 0.14 ka and 11.29 ±0.89 ka) and one 14C (1720 ± 90 Cal BP) ages from one ash layer at 58 cm in section A, and two 14C ages (1218 ±71 Cal BP and 1067 ±102 Cal BP, respectively) from another two contemporaneous ash layers in section Β and C (without distance mentioned). Finally, the ages of the ash layer in section A were replaced by the 14C age from section C, because they supposed the older OSL age (11.29 ± 0.89 ka) was contaminated or light exposed, while the 14C age (1720 ± 90 Cal BP) was overestimated due to ‘old carbon’ influence, being disordered with the OSL age (1.35 ± 0.14 ka) beneath it and older than the ash layer (1067 ± 102 Cal BP) in section C. The OSL age (6.97 ± 0.78 ka) in 274 cm in section A was also rejected because it was older than the OSL age (5.06 ± 0.50 ka) from 348 cm and the 14C age (4756 ± 109 Cal BP) from 305 cm.
For archaeological sites, cross-check between OSL and 14C ages is crucial for confirming the chronology or revealing the potential disagreement. In this study, we provide a detailed cross-checking between OSL and AMS 14C dating in aeolian sediments, some of which contain HARs, to discover the disagreement and try to discuss the mechanisms by analyzing dating materials, depositional processes, and post-depositional disturbances.
. Study Area
The QB is located at the northern margin of the QTP (Fig. 1A), with an area of 1.2 × 105 km2 and has an elevation of ca. 2800 m a.s.l. at the playa floor. The annual mean precipitation decreases from the east to the west because the precipitation is mainly controlled by the Asian Summer Monsoon (Yu and Lai, 2014), e.g. the mean precipitations of the Xiariha Town, Chahan Us Town, Xiangride Town and Nuomuhong Town in the eastern QB (Fig. 1B) was 240.8 mm, 180.5 mm, 166.8 mm and 37.9 mm, respectively, and that of the central and western QB is even lower (Chorographical Committee of Dulan County, 2001). However, annual mean evaporation of the central basin is as high as 3000–3200 mm. Due to the arid climate and strong wind from western and northwestern direction, the landforms are mainly composed of wind-erosion yardangs, salt lake playas and aeolian deposits from west to east in the basin, surrounded by alluvial and fluvial fans from the 5000± m high Qilian, Kunlun and Altun Mountains (Fig. 1B). Tiekui Desert locates in the eastern basin, while oases develop on loess-paleosol and flood deposits by the reviers around the eastern and southern desert margin (Fig. 1C).These oases are the main modern human habitat in the QB, and might have also served as a stepping stone for prehistoric people’s migration from low elevation to the hyper-arid QB and Tarim Basin, and to the colder and higher central QTP. The Nuomuhong Culture at 3400–2450 Cal BP is the most important early stage culture in this region (Cui et al., 1999; Dong et al., 2016). The large scale development of this region started since the 4th centry, when the famous “Silk Road” through the Tarim Basin was blocked by frequent wars, and Dulan became the most important transportation junction on the “Southern Silk Road” in the QB, connecting central China and arid central Asia (Cui et al., 1999, Yu et al., 2015).
. Sections and Samples
The Hongshanzui (HSZ, 3172 m a.s.l.) site locates in a gully on a terrace of the Chahan Us River (Figs. 1C and 3), the similar region with Niu et al. (2010), where HARs are widely distributed in the aeolian sediments, e.g. dozens of ash layers containing charcoals, ash and baked sheep dungs, scattered pieces of bones, and sheep dung layers. The thickness of aeolian sediments, containing HARs on the left bank of the gully, is about 1.3–1.4 m. OSL and radiocarbon dating samples were taken from three (HSZ1, HSZ2 and HSZ3) sections. HARs were found in the whole HSZ1 section, especially in two ash layers at 0.75 m and 1.2 m. Four 14C samples (two charcoals and two baked sheep dungs) and four OSL samples from this section were taken. An ash layer of ca. 3 m long outcropped at 0.4 m depth of the HSZ2 section which locates ca. 10 m north to HSZ1 section, and only one OSL sample was taken. Some dry sheep dungs and bones were found in three different layers, where three OSL samples were taken from, in the HSZ3 section, and a bone sample for 14C dating was also taken from the second layer. Besides these three sections, large amount of ash layers, sheep dungs, bones and charcoals were found but not sampled in this site (Fig. 2).
The Hebeicun (HBC, 3413 m a.s.l.) site is located at the east end of the modern Tiekui Desert, with aeolian sediments overlying on fluvial sediments (Figs. 1C and 3). There are many gullies with depths of 3–5 m around a branch of the Xiariha River. The HARs could be found along these gullies. Seven hearths, dozens of carred highland barley grains, scattered bone pieces, angular big (>1 cm3) charcoals, and several pottery sherds were found within the distance of a few hundred meters, part of which are shown in Figure 2 and also Figure 2B in Yu et al. (2015). We have studied the desert evolution and environmental changes recorded by the HBC1 section based on OSL dating (Yu et al., 2015). A cultural layer with bones and charcoals at 3.2–3.3 m, a few charcoals at 3.6 m, and a few bones at 4.0 m in the lower loess layer were found (Fig. 3). In the HBC7 section, dozens of meters to the east of HBC1 section, a hearth (0.6 m long and 0.05–0.08 m thick) was found at the depth of 1.30 m, and a potsherd, a bone and a few charcoals were found around the depth of ca. 1.50 m (Fig. 2), and another hearth (1.6 m long and 0.08–0.10 m thick), was found at the depth of 2.90 m of the bottom of the loess deposits (Yu et al., 2015). For more details of HBC2, HBC3, HBC4, HBC6, and HBC7 section please refer to Figure 3 in Yu et al. (2015).
Fig. 3
Sections, samples and ages in this study. The OSL ages in the HBC1 section are cited from Yu et al. (2015), and those in the XRH1 and XRH5 sections are cited from Yu and Lai (2014). Few OSL ages in XRH5 section are a little different from Yu and Lai, (2014) due to the recalibration of average water contents.
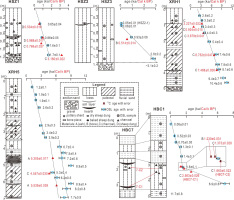
The Xiarihal (XRH1, 3174 m a.s.l.) and XRH5 (3201 m a.s.l.) aeolian sections locate on the fluvial sediments of the Xiariha River terraces (Figs. 1C and 3). Only charcoals and ash layers are frequently found in these two sections, especially the XRH 5 sections, in which charcoals and ash layers were found in more than 30 layers. These ash layers were usually thin (<5 cm) and narrow (< 50 cm), different from those in the HSZ and HBC sites. However, the frequently occurrence of charcoals and ash layers in XRH5 section might be resulted from human activities. HARs were not found in the XRH1 section, as a result this section should be the less artificially affected. 26 OSL ages have offered age control for these two sections (Fig. 3, Yu and Lai, 2014). In this study five 14C samples were taken from each of these two sections (Fig. 3), to make a comparison between 14C and OSL dating results.
16 14C samples were taken from the HARs, and to compare with them, eight new OSL samples were taken. The detailed positions of the OSL samples are shown in Fig. 3. All OSL samples were collected by hammering steel tubes (~22 cm long cylinder with a diameter of ~4 cm) into freshly cleaned vertical sections. Bulk samples were also collected in each location and sealed immediately for the dose rate and water content analysis.
. OSL and 14C Dating
OSL dating
OSL sample preparation and measurement techniques
In the laboratory, the unexposed middle part of the tube was used to extract quartz for equivalent dose (De) determination. The samples were treated first with 10% HCl and then with 30% H2O2 to remove carbonates and organics, respectively. These samples are mainly from paleosol and loess, which are mainly composed of silt, so the major fraction of 38–63 μm was extracted by wet sieving. The samples were then etched by 35% H2SiF6 for about two weeks to remove feldspars (Lai et al., 2007; Roberts, 2007), and then washed with 10% HCl to remove fluoride precipitates. The purity of quartz grains was checked by IRSL, and if the ratio of IRSL signals to OSL signals is higher than 10%, the samples have to be retreated with H2SiF6 (Lai and Brückner, 2008). The quartz grains were then mounted with a diameter of ~0.5 cm on stainless steel discs using silicone oil.
OSL measurements were made using an automated Risø TL/OSL-DA-20 reader equipped with blue diodes (λ = 470 ± 20 nm) and IR laser diodes (λ = 830 nm) in the laboratory of Qinghai Institute of Salt Lakes, Chinese Academy of Sciences. The luminescence was stimulated by blue LEDs at 130°C for 40 s, and detected using a 7.5 mm thick U-340 filter (detection window 275–390 nm) in front of the photomultiplier tube. Ninety percent diode power was used. Irradiations were carried out using a 90Sr/90Y beta source built into the Risø reader.
A preheat plateau test was conducted on sample HSZ1-4, and the preheat temperature of 220, 240, 260, 280 and 300°C was tested. The result showed a preheat plateau from 240 to 280°C, so 260°C for 10 s was chose as the preheat temperature for natural and regenerative doses, and cut-heat was at 220°C for 10 s for test doses in this study. For the younger sample HSZ2 and HSZ3, the preheat temperature was 220°C, and cutheat temperature was 200°C. Signals of the first four channels (0.64 s) stimulation were integrated for growth curve construction after background subtraction (last 10 seconds).
Equivalent dose determination
The validity of the SAR protocol was tested with a “dose recovery test” (Murray and Wintle, 2003). This test was conducted on sample HSZ1-4, and 6 aliquots were tested. The given laboratory dose was 5 Gy (approximately equal to its natural De), and the measured De was 4.8 ±0.41 Gy. Thus, the ratio of the measured to the given dose was 0.960 ± 0.082, suggesting that the SAR protocol is suitable for De determination. Recuperation was calculated by comparing the sensitivity-corrected OSL signal of 0 Gy to the sensitivity-corrected natural signal, and the recuperation was <3% for all samples. The “recycling ratio” was introduced to check for sensitivity change correction (Murray and Wintle, 2000), and for most aliquots, the recycling ratios fall into the acceptance range of 0.9–1.1.
In this study, the combination of SAR protocol (Murray and Wintle, 2000) and the Standardized Growth Curve (SGC) method (SAR-SGC method) (Roberts and Duller, 2004; Lai, 2006; Lai and Ou, 2013), was employed for De determination. For each sample, 6 aliquots were measured using the SAR protocol to get 6 growth curves which were then averaged to construct a SGC for this individual sample. Then 10–15 more aliquots were measured for the values of standardized natural signal (LN/TN) using the same measuring condition as used in the SAR protocol. Then the value of LN/TN of each of these 10–15 individual aliquots was then matched in the SGC to obtain a De. For each sample, the final De is the mean of all SAR Des and SGC Des.
Fig. 4A shows the growth curves of six individual aliquots and the SGC (red curve) for sample HSZ3-2. All the growth curves can be well fitted using the exponential plus linear function. Figs. 4B and 4C show typical OSL decay curves and components fitting of a decay curve of sample HSZ3-2, showing that the OSL signals are mainly from the fast component.
Dose rate
The concentrations of U, Th and Κ were measured by neutron activation analysis. For the OSL samples in archaeological site, there are sometimes a lot of HARs within cultural layers, e.g. charcoals and ash, which can lower the average concentration of U, Th and Κ in sediments. Due to the limited thickness of cultural layer, the effect of heterogeneous environmental radionuclide concentration on the gamma dose rate should be considered. As a result, these foreign materials should be included in the buck samples for laboratorial U, Th and Κ concentration measurement according to their natural volume ratio in the sediments. Sampling location should also be taken into account, because to take a sample inside or outside a hearth will cause underestimation or overestimation to the effective gamma dose rate from surrounding sediments, respectively. Whether this influence could be ignored depends on the volume ratio of charcoals in the sediments and their distance to the OSL samples. In most sections, the effect of human activity remains to gamma dose rate could be ignored due to their extremely low ratio in the sediments. Even in the HSZ site with more HARs, environmental dose rates are not lowered compared with those in the same depth in HSZ2 and HSZ3 sections. HSZ 1-4 sample has lower dose rate due to higher ash ratio, this will not make the dose rate underestimated because it is really close to the underlying coarse fluvial sediments which usually have lower dose rate.
The water content, a crucial changing factor for dose rate, of each sample was measured. However, due to the seasonal and historic difference of soil moisture, it’s misleading to take the measured values as the historic mean values, alternatively, we take estimated values based on the measured value, mean measured value of loess in this region, and paleoclimatic changes (Yu and Lai, 2012, 2014; Yu et al., 2015).
For the 38–63 μm grains, the alpha efficiency value of quartz was taken as 0.035 ± 0.003 (Lai et al., 2008). The cosmic-ray dose rate was estimated for each sample as a function of depth, altitude and geomagnetic latitude (Prescott and Hutton, 1994). Finally, elemental concentrations were converted into annual dose rates according to Aitken (1998). The dose rates are shown in Table 1.
Table 1
Environmental radioactivity and OSL dating results.
aaliquot number used for SAR,b aliquot number used for SGC.
14C dating
Dating materials
To make cross-check, 14C samples were mainly taken from the same layer as the OSL samples. Different dating materials were taken for 14C dating in this study, e.g., sheep dungs, charcoals, ash, and bones, so their significance for 14C dating should be analyzed for a better understanding of the results.
Charcoals are the most common materials for archaeological sites dating, and often offer good age control (e.g., Madsen et al., 2006; Hou et al., 2012, Han et al., 2014). Due to their origin of woody plants, 14C ages rep resent when the woody materials formation rather than human activities. This makes it easy to be affected by the ‘old carbon’, which originate from the internal part of old trees, old dead trees, and also the wood in ancient tombs and buildings. Moreover, charcoals are easy to be re-transported and redeposited, causing overestimation. That is 14C ages from charcoals are easy to be overestimated and seem impossible to be underestimated. The advantage of big charcoals is their original species could be identified, offering paleo-vegetation information (e.g., Han et al., 2014).
The ash, like the charcoals, is the remains of burnt plants, but it is powdery. Little charcoal pieces are usually floated from sediments for dating. As a result, it is dated as mixture of both herbaceous and woody plants, increasing the possibility of overestimation, though the herbaceous plants are not affected by the old carbon.
Animal dungs are not quite common in archaeological sites, but sometimes sheep dungs could be well preserved in arid regions. Sheep dungs used for 14C dating in this study are oval and carbonized, like the fresh sheep dungs in appearance. We assume that they have been baked in hearths; otherwise they were difficult to be preserved. They are different from ‘dry sheep dungs’, whose organic materials have been decomposed, with loess-like color and loose structure. The baked sheep dungs are suitable materials for 14C dating, because the dungs keep the same 14C/12C ratio, originated from the live grass and leaves eaten by the sheep, with the atmosphere at that time. This can avoid the contamination of ‘old carbon’, and offer the age of human activities. Additionally, being fragile, intact sheep dungs can minimize the possibility of re-transportation and redeposition, showing advantage on stratigraphic dating. Consequently, sheep dungs are ideal material for 14C dating in archaeological sites.
Bones can be dated by 14C dating with carbonate and collagen, and the latter is more welcomed. However, bulk collagen age estimates are also generally thought to be limiting ages since the proteins in collagen degrade differentially (e.g., Minami et al., 2012). That is 14C ages from bones might be underestimated.
Samples measurement
15 AMS 14C samples (charcoals, ash, bones, and sheep dungs) were measured in AMS Laboratory at the School of Archaeology and Museology of Peking University; while another two charcoal samples were dated in Xi’an AMS Center, Institute of Earth Environment, Chinese Academy of Sciences. The ash samples were pretreated to flotation the charcoal pieces for dating, while collagen were extracted from bone samples for dating. The detailed pretreat and measure processes for charcoals (including carbonized sheep dungs) and collagen follow the routine method applied in Peking University (Qiu, 1990). The radiocarbon ages were then calibrated into calendar years using the INTCAL 13 calibration.
. Dating Results and Discussion
Disturbance cause OSL and 14C ages disagreement
Results of OSL dating and AMS 14C dating are listed in Tables 1 and 2, respectively. Stratigraphy, ages, and depth-age models are shown in Fig. 3.
Table 2
Results of AMS 14C dating.
Sample ID | Lab Code | Materials | 14C Ages (14C BP) | Calender ages (Cal BP) | OSL ages (ka) and references |
---|---|---|---|---|---|
HSZ1-0.40 | BA101622 | sheep dung | 540 ± 25 | 539 ± 14 | 0.650 ± 0.04, this study |
HSZ1-0.75 | BA101623 | sheep dung | 1075 ± 25 | 968 ± 17 | 0.860 ± 0.05, this study |
HSZ1-0.90 | BA101626 | charcoal | 880 ± 20 | 768 ± 27 | 1.6 ± 0.1, this study |
HSZ1-1.20 | BA101625 | charcoal | 2975 ± 25 | 3160 ± 22 | 1.7 ±0.1, this study |
HSZ3-0.80 | BA101620 | bone | 460 ± 35 | 514 ± 14 | 0.93 ± 0.06, this study |
HBC7 | XA14483 | charcoal | 2517 ± 24 | 2563 ± 26 | no |
HBC1-3.25 | BA101621 | bone | 1245 ± 25 | 1230 ± 31 | 1.6 ± 0.1, Yu et al. (2015) |
HBC1-3.25* | BA101624 | charcoal | 1490 ± 30 | 1373 ± 28 | 1.6 ± 0.1, Yu et al. (2015) |
HBC7-C2 | XA10486 | charcoal | 1802 ± 28 | 1663 ± 22 | no |
XRH1-1.00 | BA101630 | Charcoal | 3030 ± 25 | 3227 ± 22 | 3.3 ± 0.2, Yu and Lai (2014) |
XRH1-1.20 | BA101631 | charcoal | 995 ± 25 | 924 ± 15 | 3.4 ± 0.3, Yu and Lai (2014) |
XRH1-1.60 | BA101632 | charcoal | 3435 ± 30 | 3679 ± 41 | 4.0 ± 0.3, Yu and Lai (2014) |
XRH1-3.00 | BA101633 | charcoal | 5925 ± 40 | 6752 ± 47 | 7.4 ± 0.6, Yu and Lai (2014) |
XRH1-3.95 | BA101634 | charcoal | 6605 ± 35 | 7488 ± 26 | 7.9 ± 0.6, Yu and Lai (2014) |
XRH5-4.65 | BA101628 | ash | 4575 ± 35 | 5305 ± 17 | 7.2 ± 0.6, Yu and Lai (2014) |
XRH5-5.55 | BA101627 | charcoal | 4090 ± 25 | 4557 ± 30 | 5.3 ± 0.4, Yu and Lai (2014) |
XRH5-6.25 | BA101629 | ash | 5295 ± 40 | 6079 ± 39 | 8.2 ± 0.8, Yu and Lai (2014) |
Lab codes with BA is dated In Beijing University, while XA mean from Xi’an.
In the HSZ1 section, both OSL and 14C ages increase with depth and the upper two pairs of ages are in agreement. However, the lower two 14C ages disagree with the OSL ages, especially the lowest one (3.160 ±0.022 Cal BP vs. 1.7 ±0.1 ka). This makes it confuse that when did the aeolian sediments accumulation and human activities start. Was this caused by the overestimation of 14C age or the underestimation of OSL age? Age-depth model based on OSL ages shows stable accumulation rate in HSZ3 section, suggesting that the OSL ages should be reliable. According to the age-depth model, loess should start at ca. 3.1 ± 0.2 ka, which agrees with the 14C age of 3160 ± 0.22 Cal BP in HSZ1 section. As a result, human activities occurred in this site at ca. 3.1 ka, corresponding to the Nuomuhong Culture (Cui et al., 1999; Zeng, 2006; Yu et al., 2015; Dong et al., 2016).
There are many other disagreements on ages, e.g., the 2nd and 3rd 14C ages in HSZ1 section are disordered, and the 14C age of a bone (514 ± 14 Cal BP) is ca. 400 yr younger than the OSL age (0.93 ± 0.06 ka) 0.5 m away in HSZ3 section. Usually, the 14C ages from charcoal should not be underestimated, while sheep dung samples should not be overestimated. Consequently, the occurrence of older sheep dung age (968 ± 17 Cal BP) in younger stratigraphy (Fig. 3) in HSZ1 section should be only resulted from disturbances, and all the other aforementioned disagreements could be also interpreted by disturbances. Additionally, though HARs occur frequently in any depth in this region, 14C ages which are more directly associated with human activities seem mainly centralized in three periods i.e. 560–615, 800–1045 and 3140–3180 Cal BP.
OSL age at 40 cm of the HSZ2 section is 0.05 ± 0.01 ka, similar with HSZ3-1 (0.06 ±0.01 ka) from 50 cm depth in the HSZ3 section. This repeatability of the young ages shows the OSL dating is suitable for the young samples in the archaeological site. These two ages are much younger than that from the same depth in the HSZ1 section (ca. 0.6 ka, ~10 m away to the south of the HSZ2 section), implying the complexity of chronology in archaeological sites that ages from the same depth in two adjacent sites might have very different ages.
The anthropogenic layer at the depth of 3.2–3.3 m in the HBC1 section was dated to younger than 1.6 ± 0.1 ka by OSL dating (Yu et al., 2015), and 1373 ± 28 Cal BP (charcoal) and 1230 ± 31 Cal BP (bone) by 14C dating. Age-depth model suggests that OSL chronology of HBC1 section is reliable; and 14C age from charcoal agree with this model. Moreover, according to the comparison between cultural layers in HBC1 and HBC7 sections, 14C ages of charcoals in HBC7 section (1663 ± 22 Cal BP and 2563 ± 26 Cal BP) also agree with the age–depth model from the HBC1 section. As a consequence, 14C age from the bone in HBO section should be underestimated.
Chronology of the XRH1 section has been well established by ten OSL ages in Yu and Lai (2014). Five charcoals were dated by 14C dating in this study, and four of them could be well compared with the OSL dating result, except the one at the depth of 1.2m (0.924 ± 0.015 Cal BP vs. 3.4 ± 0.3 ka). This charcoal is very tiny (<2 mm), the cause for the serious underestimation is unknown. Without much anthropogenic disturbance, the age-depth model based on both OSL and 14C ages show stable accumulation rate of aeolian sediments.
The XRH5 section has also been dated by 16 OSL ages (Yu and Lai, 2014). Age-depth model based on OSL ages show consistent depositional rate in the upper section, however, both OSL and 14C ages are disorder in the lower section. Tow age-depth models could be identified, with similar depositional rate but different ages. The younger model based on both OSL and 14C ages seems consistent with the upper section, however, the disorder of 14C ages is difficult to interpretation, because the younger 14C age (4567 ± 30 Cal BP) based on ash should not be underestimated. Consequently, the sediments should have been seriously disturbed. For the OSL ages, the age-depth model is neither robust, the two older OSL age (8.9 ± 0.5 ka and 8.9 ± 0.4 ka) from thin sand layers might be caused by the underestimated dose rate, and possible insufficient bleaching, while the two younger ones (5.3 ± 0.4 ka and 6.2 ± 0.5 ka) might be due to the bleaching caused by disturbance. According to the 14C ages in the lower section, human activities exited during ca. 6.2–4.6 Cal BP, which might have cause severe stratigraphic disturbance and also the hiatus during 6.7 ± 0.4 ka to 3.9 ± 0.2 ka at the depth of 3.6 m.
Influences of human activity disturbances on the dating results
Influence from stratigraphy disturbances
Murray and Olley (2002) reviewed comparisons between OSL ages and 14C ages or other independent ages and found most of them could be well compared, especially for the ages from aeolian sediments. This conclusion is also proved by ages from the XRH1 section in this study, in which no direct human activity evidences were founded. However, it is obvious that anthropogenic disturbance is important for chronologies in archaeological sites. In the HSZ1 and XRH5 sections, the 14C ages are obviously younger than the OSL ages, and disorder occur on both OSL and 14C ages due to the stratigraphic disturbances. Influences increase with the frequency and intensity of human activities. In the archaeological sites, human and livestock activities could usually disturb the stratigraphy, especially for the surficial sediments within 0.3-0.5 m. Stratigraphic disturbances could mix sediments from different depth unequally, due to the insufficient bleaching, OSL ages may show underestimation or overestimation in different layer compared with their original stratigraphie ages. Disturbances can also change original location and depth of the carbonaceous materials synchronously, and in this case, the 14C ages can represent the ages of the burning events (plants or animals dead), but not the ages of the surrounding sediments. As a result, stratigraphy disturbance should be the major cause for the disagreement between OSL and 14C ages in the archaeological sites. In this case, accumulation processes and post-depositional artificial disturbances should be analyzed. However, these problems are usually difficult to be found if without detailed chronological studies, or are ignored. The disagreement between OSL and 14C ages and disorder of ages in the HSZ region by Niu et al. (2010) should be also caused by anthropogenic disturbances. Additionally, disturbances from cave animals are also possible in this arid region, which is not discussed in this study.
Influence from heating events
When people use fire on the ground, hearths surrounded by stones were usually built, like those in the Qinghai Lake Basin (Madsen et al., 2006; Rhode et al., 2007; Sun et al., 2012). They also could be only simple pits on the ground, like those in the QB (HBC7 section in this study), in the Guanting Basin (Hou et al., 2012), and on the boundary between the QTP and the Tarim Basin (Han et al., 2014). Their surrounding sediments could be heated and cause luminescence signal resetting by fire, making it suitable for luminescence dating. However, many questions are associated with the dating of heating event and surrounding sediments with OSL and 14C dating, e.g., whether a pit was dug (sediments age of the ground), whether it was used temporarily or permanently, whether it has been buried naturally or artificially (maybe unbleached), etc. These questions decide the stratigraphie disturbance intensity and heat resetting depth. In this section, the temporary outdoor hearth within loess as usually found on the QTP, which is crucial for the studies of early peopling the QTP, is focused. We discuss the possible influence of fire on the results of OSL and 14C ages from different positions in and around a hearth.
a. Possible influence from heating events
During the building and abandon of a hearth, firstly, a pit was dug on the ground, and then fire was set, leaving an ash layer, and finally, the fire and ash was covered to extinguish the fire. During this process, the sediments around the hearth were baked by the high temperature.
In this case (Fig. 5), the 14C sample from the ash layer represented the time of the human activity, while sample OSL1 in the same depth with the hearth represented the real age of the original stratigraphy (0SL1>14C). Sample OSL2, from the surface of the original ground floor, should be similar with the age of 14C (OSL2~14C). Sample OSL3, very close to the ash layer, was baked by the fire, as a consequent, its OSL signals can be totally reset by the high temperature, and its age should be the same with 14C (14C=OSL3). However, this heat reset layer might be very thin. Sample OSL4 was close to the ash layer but the temperature was not high enough to reset the OSL signal, so it could be the oldest age. Then the hearth was covered artificially to extinguish the fire, so the sediments from the cover layer were poorly bleached, and the age of OSL5 should be younger than OSL1 while older than OSL2 (OSL1>OSL5>OSL2). After that hearth was covered by post natural deposition, and the sample OSL6 from the overlying sediments should be the youngest. Therefore, the sequence of these ages should be: OSL4>OSL1>OSL5>OSL2≈OSL3=14C >OSL6.
Fig. 5
Influences to the OSL and 14C ages from different positions in a hearth from the heating event.
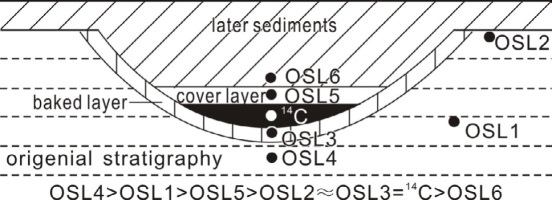
b. Field hearth experiment
In consideration of the poor thermal conductivity of the sediments, how many surrounding sediments could be heated sufficiently enough to reset the luminescence signals is a fatal question. To examine the influence of heating events on OSL signals in the surrounding sediments, an experiment was carried out in the field (Fig. 6). Firstly, a pit of 30 cm depth was dug on the loess deposits to make sure the underlying sediments have enough natural OSL signals. Secondly, fire was set in the pit and kept burning strongly with flames of about 2 m high for four hours, and a whole tree was burnt. The hearth with 10-cm-thick burning charcoals was fully buried artificially for a whole night over 12 hours. When we recover the hearth the next morning, the charcoals were still smoking, and the underlying sediments were burnt to red and too hot to touch directly. We are sure this heating experiment is more than enough to represent a temporary outdoor archaeological hearth on both burning intensity and duration. However, only the upper 13-cm-thick sediments were backed to dry, while those beneath were still humid, which mean that they were only heated to dozens of degrees centigrade. This reminds that even if for a permanent outdoor hearth, the underlying sediments can get moisten after each short using, which can limit the heating effect. Finally, the pit was cleaned and OSL samples were taken by 3-cm-diameter tubes from 5 cm (TL1), 10 cm (TL2), 15 cm (TL3), 20 cm (TL4), and 30 cm (TL5) beneath the hearth (Fig. 6) to check the influence on OSL signals. Sample TL0 was taken from the same depth as the hearth base beside the hearth, which was not affected by the heat, to show the nature signals of the original sediments. The OSL samples were pretreated following the aforementioned method. OSL signals in Fig. 7A show that the OSL signals were not reset in all these samples, implying that the temperature was not high enough. In another experiment on TL5 sample, which was not affected, TL with different temperatures were applied before stimulated with OSL, to check influences resulting from different heating temperatures. The results display that after heated to over 300°C, residual OSL signals decrease with increased preheat temperature, and are totally reset before 400°C (Fig. 7B). Then TL signals were tested to check what temperature the sample was heated to. The results show that TL signal intensity increase with the depth, and even the sample from 5 cm beneath the hearth has TL signals starting from ca. 200°C (Fig. 7C), demonstrating that these samples were not heated to over 200°C. As a result, OSL signals cannot be reset. The poor reset of the TL/OSL signals is mainly because the heat is difficult to be conducted downward into moist sediments, and also due to the poor thermal conductivity of the sediment. The OSL age (16.6 ±2.1 ka) from underlying (20 cm beneath) sediments is much older than culture layer (2.6 ± 0.2 ka and 2227–2407 Cal BP) in Erfang site in the northeastern QTP (Hou et al., 2012), offering an example of unreset sediments beneath culture layer in the field.
This remind us that if 14C ages not match OSL ages under a hearth, it might be due to the OSL signals were not reset to represent the heating event. Worse still, the possibly disturbed OSL ages should also be cautioned to use as the stratigraphic ages. As a result, when dating a hearth, it’s not advisable to take OSL sample beneath it. This is to raise the potential problems, but not to deny the possibility to date hearths with OSL. If the hearth was built by stones directly on the ground surface without artificial or natural erosion, i.e., the age of human activity equals to that of the ground surface, these influences could be minimized. The hearth in Sun et al. (2012) might have offered an ideal example for this case. On the contrary, artificial erosion mainly result from digging hearth (maybe limited by environment without stones) which are quite familiar on the NE QTP with crescentic ash layers as shown in Fig. 2. The long-term used indoor hearth is another exception which will not be discussed here.
Implications of 14C and OSL ages in archaeological sites
The disorder of 14C ages in HSZ1 section implies that the age-depth model based on 14C ages is not reliable. The 14C ages only represent human activities in two periods, but nothing with the ages of stratigraphy. OSL ages in the HSZ3 section, which are more reliable due to fewer disturbances, indicate the OSL ages in HSZ1 section are neither reliable due to the severe disturbance and insufficient bleaching. The only implication of OSL ages in HSZ1 section is to display the influence of disturbances. In the lower HBC5 section, the left younger age-depth model based on 14C ages represent the age of human activities again. Their disorder and much younger than OSL ages imply that the stratigraphy was severely disturbed, and consequently, neither of the age-models can represent the ages of the stratigraphy.
These disagreements between 14C and OSL ages demonstrate their different implications in archaeological sites. Usually, 14C ages can represent human activities, if not affected by old carbon. 14C ages from different material can help to identify the disturbances and to limit the age ranges. OSL ages represent ages of the sediments in sections with fewer disturbance. However, it’s also difficult to date the sediments with OSL dating in sections with intensive disturbance. Though influenced by underestimation, OSL ages should still close to the original stratigraphic ages, and difficult to represent human activities, even if intensively affected as demonstrated in HSZ1, XRH5, and Figs. 5 and 6. Consequently, 14C ages display human activities, while OSL ages are usually residual ages of disturbed sediments. Only in few cases, they show agreement, e.g., in temporary sites (e.g., Sun et al., 2012) or in long-term sites with low accumulation rate or sufficient disturbances and bleaching (e.g., Hou et al., 2012). In fact, the five 14C ages are all younger than the OSL age in Hou et al. (2012), even within the larger error bar. The disagreement is not quite often discovered also because OSL ages have larger error bars which can cover the age differences in older sites. The age difference of a few hundred years in HSZ1 section will be ignorable for a 10 ka site with the increase of errors of OSL ages.
The different implications of OSL and 14C ages are crucial for both archaeological and paleoenvironmental studies. Firstly, if archaeological sites are aimed to dated, directly dating on HARs and/or fossils (e.g., ESR and U-series dating on teeth) should be suggested, because indirect dating of sediments might be overestimated due to disturbance, especially for old sites without proper dating methods or materials for cross-checking. Secondly, neither OSL nor 14C is suitable for dating of sediments to study paleoenvoronmental background reconstruction in archaeological sites due to disturbances.
. Conclusions
Both OSL and 14C dating are suitable for the dating of aeolian sediments, but disagreements occur quite often in archaeological sites in the QB. The disagreements were caused by the anthropogenic disturbances to the sediments and HARs, which can mix sediments (OSL) and HARs (14C) in different depth. Field hearth experiment revealed that even intensive fire events cannot reset luminescence signals in sediments 5-cm-depth beneath the hearth. As a consequence, both stratigraphic disturbances and using fire are difficult to totally reset the OSL signals in surrounding sediments. This is the reason why OSL ages usually older than the corresponding 14C ages in archaeological sites.
In archaeological sites, OSL and 14C ages seem display different implications, i.e., the 14C ages are directly connect with human activities, while the OSL ages are still close to the original sedimentary ages though affected by disturbance. That is the indirect ages from sediments are difficult to represent human activities precisely, which demonstrates the importance of the choices on dating methods and materials. This also reminds the potential risk that many old archaeological sites dated by sediments might have been overestimated if the sediments had been severely disturbed. As a result, crosscheck between 14C and OSL dating is essential for the studies of archaeological sites to find the potential problems. If their results are disaccord, possible post-depositional anthropogenic disturbance should be taken into account.