. Introduction
Since the terrorist attacks of 9/11 there has been a heightened emphasis on National Security in general and increased interest in ‘as found’ materials for retrospective dosimetry purposes. Common salt (NaCl) has been studied extensively for its luminescence properties and more recently evaluated as a retrospective dosimetry material. Early work was summarised by (McKeever, 1984) including referencing the attribution of the 590 nm emission to de-excitation of a Mn2+ impurity from 4G to 6S state (López et al., 1977) and the attribution of the blue 420 nm TL emission to an impurity perturbed intrinsic 400 nm emission due to the combination of F and H centres in the crystal matrix (Delgado and Alvarez Rivas, 1982). Subsequent reports include characterisation of blue stimulated OSL (Bailey et al., 2000, Thomsen et al., 2002), UV (360 nm) TL (Rodriguez-Lazcano et al., 2012), blue (420 nm) TL (Gartia et al., 2004), orange (590 nm) TL, blue stimulated OSL, IRSL (Spooner et al., 2011), TL kinetics (Gartia et al., 2012), 590 nm TL and fading performance (Hunter et al., 2012). In general it has been shown that luminescence suitable for dosimetric purposes has been found in a wide range of NaCl samples (Spooner et al., 2011; Timar-Gabor and Trandafir, 2013; Gartia et al., 2004; Murthy et al., 2006; Hunter et al., 2012; Christiansson et al., 2014).
Many examples of salt crystallised in solar ponds exhibit an enhanced 590 nm TL peak, typically an order of magnitude larger than any 420 nm and 360 nm TL emissions (Spooner et al., 2011, 2012; Hunter et al., 2012). In comparison, samples that were mined from rock salt have very much weaker 590 nm emissions, if present (Hunter et al., 2012).
In order to accommodate these variable characteristics into a standardised processing protocol the question of how the manufacturing process steps, as applied to a common feedstock to produce commercial salt products, affects the dosimetric performance of the resulting salt should be investigated. This study investigates a series of salt samples taken from various stages of the manufacturing process used by Cheetham & Co., Australia. The process is typical of salt crystallisation from sea water using solar energy to evaporate the water, leaving salt and other compounds as the result. We compare the response of the 590 nm, 420 nm, 360 nm TL emissions and the UV OSL signals induced by a beta radiation dose in the range 1–5 Gy with the aim of detecting changes in luminescence properties due to manufacturing process. It is reasonable to expect the luminescent characteristics be closely correlated, with any variations due to the effect of process step(s), for example washing, heating, grinding, etc.
. Material
Salt Production
Common salt produced in Australia is sourced predominantly from sea water, saline lakes or saline ground water from salt reclamation schemes, which aim to remove salt leached from the soil from irrigation runoff water before being returned to the environment. Cheetham & Co have been producing salt using solar evaporation pans for over 100 years and operate in most states of Australia, producing a wide range of products.
The prerequisite for solar production of salt from seawater is a clean source of seawater, sufficient flat land, preferably located on the seashore, and a suitable climate viz. warm to hot temperatures with long hours of sunshine and low rainfall (resulting in a high annual evaporation rate). Suitable geographic locations on the South Australian coast and the mid to lower coast of West Australia are blessed with a Mediterranean climate with abundant sunshine, warm to hot temperatures for much of the year and rain predominantly in the cooler months.
The production facility essentially consists of a series of shallow, inter-connected ponds through which the seawater transits. As water evaporates and the salt concentration increases the brine is transferred to the next in the series of ponds before the seawater is replenished. The concentration of the brine in each pan is maintained within specified limits depending on the location in the process and, as it increases stepwise, various salts preferentially precipitate out of the brine. Relatively insoluble salts precipitate in the first pans (carbonates and calcium sulphate), followed by NaCl (the generally desirable main product) followed by more soluble salts (potassic and magnesium salts) in the later pans. The waste water left at the end of the process (“bitterns” so named from the bitter taste imparted by MgCl) is separately harvested for MgCl or returned to the sea. The water temperature is maintained at 20°C throughout the entire process with the end result being a product which is ~98% NaCl with minor impurity elements derived from the source seawater, which sometimes playing a significant role in luminescence. For example, manganese in its various aggregations and ionisation states is the predominant source of the 590 nm emission (López et al., 1977, Aguilar et al., 1978) despite only just being detectable in the samples tested here (Appendix A – salt assays).
When sufficient salt has been precipitated on the bottom of the pans the brine is drained and the salt is scraped into mounds (from which we obtained sample #45) where it is further rinsed with brine to remove surface impurities (from which we obtained sample #6) and then centrifuge dried to a moisture content of ~2%. It may be used as is, where a coarse grain size is acceptable, or further rinsed, kiln dried to a moisture content of ~0.5%, ground, graded by size, packed and sent to market (from which we obtained samples #2, #4 and #5).
Sample Details
Samples were purchased locally as a commercial product after which up to 10 g of the salt sample was placed in a closed vial stored within a desiccator to prevent deterioration of the salt crystals due to absorption of atmospheric moisture. All samples were produced in white light conditions with no signal of formation found in samples #2, #4, #5 and #6 as previously reported (Hunter et al., 2012).
Salt Samples
Salt samples #2, #4, #5, and #6 were amongst samples previously reported by (Hunter et al., 2012) where the 3D TL spectra were presented as well as the dose response, and were sourced from commercially marketed salt (bulk packaged table, cooking salt) and sachets from a restaurant. Sample #45 was subsequently obtained from the manufacturer in 2013 and is the input feedstock used in the manufacturing process. The “as received” individual sample details are as follows:
Sample #45 (feedstock): was supplied directly from the crystallisation pan and as such was in the concentrated brine, the crystal size ~5–6 mm. Over the course of a year the salt was air dried at laboratory temperature with the crystals being “welded” together by the brine as it crystallised. It was not subjected to heating above ambient room temperature.
Sample #6 (Diamond) was marketed as water softener salt and was supplied as coarse crystals of ~5–6 mm diameter and subject to some amalgamation in the packaging but with crystal size of the order of the feedstock.
Samples #2, #4, #5 were all processed by the manufacturer in a similar manner, being double washed, kiln dried for ~5 minutes @135°C to give a moisture content of ~0.5 %, ground, graded by size and packed for distribution.
Sample # 2 (Saxa)- cooking salt which had a received grain size ~150 µm with sodium ferrocyanide added as an anti-caking agent.
Sample #4 (Coles) - home brand table salt marketed by Coles supermarkets in 1 kg packages. It has received grain size ~100 µm in size and has sodium aluminate added as an anti-caking agent and fortified with potassium iodate to help ensure adequate iodine in the diet.
Sample #5 (ISM)- sachet salt sold to local cafes for use as a condiment and since it is sold in single serve packs is ground to a smaller size, ~100µm, ensuring it will dissolve in the food when used. No additives were listed on the salt packaging.
. Sample Preparation
After receipt of a sample, it was gently hand ground in an agate mortar and pestle and sieved for the 180–250 μm fraction; the 125–180 μm fraction was also utilised for small batches, e.g. salt supplied in sachets typically containing <1 g of salt. The samples were mounted on 9.7 mm diameter stainless steel discs with silicone spray as an adhesive and masked to the central 7 mm area. Finely ground salt has a tendency to “clump” together and cause some significant variation in mass between discs so mass normalisation was carried out in all cases. However this normalisation still left a residual ~7% variance in the results, which did not correlate with mass or dose.
Significant sensitivity changes in the OSL (Zhang et al., 2005) and 590 nm TL (Spooner et al., 2011) reported when recycling salt aliquots mandated the use of the first glow only, with a reheat run to subtract the background incandescence from the TL glow curves.
Apparatus
Spectral measurement was carried out using the 3D TL spectrometer (Prescott et al., 1988) which was developed in a series of research projects and is a manually operated TL system with automated software modules that ensure a consistent approach to apparatus operation and record taking. It is a single aliquot apparatus where each step of the process (sample loading, chamber evacuation and TL testing) is initiated by the operator, requiring their constant operational presence. To minimize operator time, it is therefore preferable that only one aliquot of each sample be processed for each step in a project under the assumption that multiple aliquots of the same sample will give essentially identical results. The test parameters used were 5 Gy beta dose using a Daybreak irradiator with 40 mCi 90Sr/90Y plaque β source followed by immediate glow to 400 °C @ 5 °C/s heating rate, reheat for background subtraction and PMT quantum efficiency correction. The results for Saxa, Coles, ISM and Diamond salt samples were previously presented as contour plots of the TL emissions by Hunter et al., 2012 as a part of a larger sample study of NaCl luminescence. An order of magnitude of the luminescence intensity (z axis) is represented by the displayed contour lines, referenced to the peak emission intensity (Hunter et al., 2012). The 3D TL spectrum of the feedstock is presented here for the first time.
Comparative testing of the separate TL emission bands (single aliquot only per sample) and OSL response (two aliquots per sample) was carried out for the TL signals at 360 nm, 420 nm and 590 nm and the UV OSL using a Riso TL/OSL DA-20 reader. Beta irradiations were administered using a 1.5 GBq 90Sr/90Y plaque, delivering 0.105 Gy/s, and luminescence was detected using an electron tube 9235QB bi-alkali PMT. Optical stimulation was performed using LEDs emitting at 490 nm. A 20 second dose (at 0.105 Gy/s) was applied from the internal beta irradiator to each sample aliquot in turn and luminescence read out almost immediately. All TL measurements were made after evacuation of the chamber to 7 Pa and then flushing of the chamber with dry nitrogen gas, to prevent spurious TL emissions.
All TL measurements were made to a maximum of at least 450°C, at a heating rate of @1°C/s for 590 nm and 360 nm TL and 5°C/sec for 420 nm TL. The signal was summed over the temperature range ~200–400°C, with the lower temperature set to ensure the low temperature emission peaks were as completely as possible excluded without impacting on the higher temperature peaks. Each aliquot reheat was subtracted to eliminate the background incandescence, and intensity correction was made to reflect PMT quantum efficiency response. This is important for quantitative sensitivity comparison, as there is a lower quantum efficiency for the 590 nm signal (15%) and the 420 nm signal (85%) compared to the 360 nm response, which was taken as unity.
OSL measurements were made at 100°C for 100 sec. after preheating to 160°C for 10 s to remove the short lived luminescence signal originating from shallow traps. The total light sum was used with the background (average of the 90–100 s) subtracted.
For all OSL and TL testing a fused quartz window was used in addition to 7.5 mm HOYA U340 filter for OSL, 3 mm Schott glass OG530 for 590 nm TL (passing wavelengths greater than 530 nm), Corning 4 mm 7–59 band-pass filter and 3 mm Schott glass GG420 filters for 420 nm TL (giving a pass band of 400–450 nm) and 4 mm Schott glass UG11 filter for 360 nm TL (passing wavelengths less than 380 nm).
. Experiment and Results
All OSL and TL results are mass and dose normalised to account for mass variations between aliquots and differing doses between some data runs and have units of “counts/Gy (mass normalised)” abbreviated to: cts/Gy(mn).
Measurement Reproducibility
Emission Spectra
Given that the operator time required is in excess of fifteen minutes per sample, it is desirable to process only the minimum number of aliquots to ensure reliable results, ideally one aliquot per sample. The spectrum of Diamond salt previously published (Hunter et al., 2012), (reproduced here for convenience as Fig. 1a) and the spectrum measured in this project (Fig. 1b) were observably different, which was unexpected because the sample and measurement protocol were identical differing only in that they were made four years apart.
Figure 1
Diamond Salt — First Glow. (5 Gy dose, heating rate 5 K/s). Left — Hunter et al., 2012 Right — present study
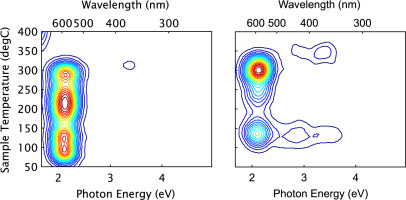
Reproducibility between individual aliquots of the 3D TL spectrometer results was investigated and confirmed by measuring the spectrum of five identically prepared aliquots of Diamond salt for the first glow, with a reheat for background subtraction.
The series of five identically tested aliquots of Diamond salt gave effectively identical 3D TL plots with the 590 nm band displayed within 5 nm and the dominant TL peak separation being 150–160°C despite the peak’s inter-aliquot variation by 30°C, possibly due to thermal lag effects (Fig. 2).
Second Glow Characteristics
Bearing in mind the justification for utilizing only the first glow TL, measurement reproducibility results were extended with a further irradiation/glow measurement cycle.
These second glow spectra likewise presented a consistent picture across all 5 aliquots with the 590 nm ~140°C peak remaining roughly constant and the ~280°C peak decreasing by ~ order of magnitude compared to the first glow spectrum (Fig. 3). Conversely UV emissions of 360 nm wavelength were enhanced, the 130°C peak especially so, as indicated in a previous study (Spooner et al., 2012).
Variance after artificial dosing
Two groups of five aliquots of feedstock were identically irradiated and processed to observe the 590 nm and the 360 nm emission bands. For a dose of 2 Gy the intensity was measured, the variance in results calculated and recorded in Table 1. The 590 nm TL emission for group 1 were recorded and similarly the 360 nm TL emissions for group 2. In both cases the result variance was less than ±7% from the mean value.
Sample Survey
3D TL Spectra
The spectra for samples #2,#4,#5 and #6 were previously published by (Hunter et al., 2012) and are presented here for ease of interpretation with the spectrum for feedstock( #45) which is presented here for the first time.
The 3D TL plots for the five study samples display a consistency in emission wavelength and TL peak temperatures, attributed to their common origin (Fig. 4)
Figure 4
Salt Survey. Luminescence Intensity vs Photon Energy and Temperature. (5 Gy dose, heating rate 5 K/s).
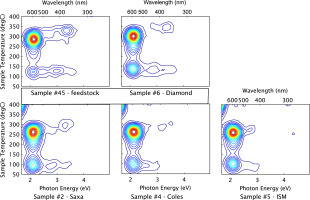
The results clearly indicate that the 590 nm (2.1 eV) emissions are dominant in all the sample aliquots, having peak maxima at ~100°C and ~260°C for the 590 nm signal and some detectable 420 nm (2.9 eV) and 360 nm (340 eV) signals.
TL and OSL intensities for the survey samples
The intensity of the luminescence emission bands at 590 nm, 420 nm and 360 nm was measured for each sample and is presented in Fig. 5. The values shown are the light sums over the range ~200–400°C (to select the ~270°C dosimetric emission peak).
These results are presented roughly in the order of processing: –feedstock (#45), Diamond (#6)) – double washed only and then the remaining, more processed samples which were kiln dried and crushed and graded by size and packaged for distribution. It may be expected that the feedstock and Diamond display different luminescence characteristics due to lack of processing (heating, crushing) compared to the other samples. We observed the processed samples to have a 55% higher 590 nm emission, but ~40–70% lower emission at the other wavelengths except the feedstock UV TL which is anomalously high and all the foregoing requiring further investigation.
The UV OSL signal is believed to be derived from the same centres as the high temperature (~340°C) 360 nm TL signal (Bailey et al., 2000) and both of these emissions are observed here to correlate with each other in intensity with the OSL being ~50% of the UV TL signal across all the samples, except the feedstock as noted above, for the test parameters used.
Heat Sensitization of 590 nm emission
Further sensitisation experiments were conducted to investigate any potential effect on 590 nm emission intensity due to the drying step in the production process. Two groups of five aliquots each of feedstock were loaded, where the first group was irradiated and TL measurement without preheating whilst the second group was preheated to 135°C for 5 minutes before irradiation and TL measurement of the 590 nm emission. Total light sum in the range ~200–400°C was counted for all aliquots, mass and dose normalised with the results averaged for all the aliquots in each group (Table 2).
Table 2
Sensitization from 135°C preheat of feedstock. Light sum (200–400°C) for 590 nm emissions.
After the 135°C preheat, the 590 nm emission intensity increased by 10%, which is outside of the one standard deviation calculated for each group, so no sensitization was observed here
. Conclusions
As suggested by the 3D-TL spectrometer spectra presented by (Hunter et al., 2012), the results for the individual 590 nm, 420 nm, 360 nm Tl and blue OSL emission intensities were found to be consistent with the hypothesis that there is minimal effect on the balance of luminescence characteristics caused by the manufacturing processes used in the manufacture of these products.
Investigating the effect of heat treatment of unirradiated, unheated sample material (feedstock) showed only slight (10%) increase in 590 nm emission intensity due to the pre-heat treatment, which did not accord with the larger observed change in sensitivities between the un-heated (feedstock and Diamond) and heated samples (Saxa, Coles and ISM), of the order 50%. Further work is required to resolve this anomaly as well as the high UV TL emissions from the feedstock compared to the other samples.
Diamond salt showed strong 590 nm emissions and some 420 nm and 360 nm emissions. Second glow spectra, whilst differing from the first glow spectra, had a similar consistency between aliquots.
We conclude that when the salt samples are manufactured on the same production line they have similar luminescence characteristics, although some slight sensitivity changes may occur, and so are amenable to evaluation using a standardized testing protocol.