. Introduction
The stimulated luminescence properties of natural quartz, the most reliable natural phosphor, have been widely used in variety of applications in general and retrospective dosimetry and dating of sediments in particular. Quartz grains, once irradiated, naturally or artificially, release luminescence by stimulation with heat or light in the visible range. The emissions due to thermal and optical stimulations result in thermoluminescence (TL) and optically stimulated luminescence (OSL) respectively. These emissions occur due to recombination of charge released from the traps due to thermal or optical stimulation with the luminescence centre. Quartz has been observed to have a variety of traps, ranging from shallow to deep, which participate in stimulated luminescence process in some way or other (Sankaran et al., 1983).
The OSL emission of quartz has been found to possess in general three components (fast, medium and slow), though more than three components have also been reported in literature (Jain et al., 2003; Singarayer and Bailey, 2003). Unlike the TL process, the traps are not emptied serially with the optical stimulation and therefore, activation of charge from more than one trap simultaneously might be possible, albeit with varying cross-section. The initial, fast, OSL of the decay curve is believed to be associated with the 325°C TL glow peak. This has been proposed by Smith et al. (1986) and subsequently supported by the experimental work of Smith and Rhodes (1994) and Wintle and Murray (1997). The saturation of this emission has been reported to occur at around 100 Gy (Murray and Wintle, 2000; Godfrey-Smith et al., 1988).
Studies carried out to see the thermal stability of different OSL components of quartz showed the removal of both, the fast and medium component with preheating at higher temperatures, around 400°C (Jain et al., 2003; Singarayer and Bailey, 2003). The component of OSL observed to survive with such high pre-heating temperature was found to be only the slow component Singarayer and Bailey, 2003; Bailey, 2000). The nature of bleaching of fast and medium components was seen to be similar with different stimulation photon energies (Singarayer and Bailey, 2003). The ratio of the fast to medium emissions decreased with increase in stimulation energy; varying from 30.6 at 590 nm to 1.4 ± 0.3 at 375 nm. These components have been observed to get reset with an exposure to sunlight of few seconds and, so, both components contribute to the initial part of the OSL signal that is generally used in dose assessment Murray and Wintle, 2000). Based on the component analysis of the CW-OSL curve, Wintle and Murray (2006) found the fast and medium components to decay completely within 10 and 40 s of bleaching with sunlight respectively. On the other hand, it could take as much as two weeks of exposure to sunlight to bleach out the slow component completely (Bailey, 2000).
Thermal treatment, firing, has been reported to have a profound influence on the luminescence properties of quartz (Schilles et al., 2001). The general observations reported by various workers are: (i) variation in TL sensitivity (Li, 2002, Polymeris et al., 2006; Koul and Chougaonkar, 2011), (ii) formation of new TL glow peaks, (iii) shifting of the TL glow peak positions and (iv) change in the TL spectral emission. The competing centre, E1/- centre, has been observed to get annealed out with firing, the process starting at ~ 300°C and reaches completion at ~ 700°C (Benny et al., 2002). This phenomenon of reduction in the competition during read out stage has been proposed to be a major factor for the sensitization of the luminescence signals. Also, the composition of the signal has been found to get disturbed with firing, slow component reducing significantly (Koul et al., 2016).
The survival of the OSL decaying faster than the slow component, with pre-heating at such high temperatures (> 400°C) was reported recently by Koul et al. (2014) in three of the four fired samples studied in their work (Koul et al., 2014). The Greek samples, in particular, yielded dominant signals with high pre-heating temperatures. The results, especially, the nature of the pulse annealing curves, in this work did suggest some contribution of thermally transferred OSL (TT-OSL) (Adamiec et al., 2010). It was this process which was proposed to sustain the OSL with higher pre-heating temperatures. However, this phenomenon could not explain the existence of OSL which can be associated with this trap at pre-heat as high as 500°C.
The work reported in this paper tried to investigate the post 500°C blue stimulated OSL emission of fired quartz specimen. This signal was seen to exist in all the samples studied in this work, a local brick sample, Indian dessert sample (Thar), geological Greek sample (Koupa) and Polish sedimentary sample. Subsequently, one of these samples, namely the Greek sample, studied in detail in this work, acted as a representative sample of all the samples which yielded this signal. The deep trap responsible for the post-500°C post-blue OSL has been identified and characterized. The observed signal was found to be dominated by initial medium component. The trap was found to be bleachable, the signal getting bleaching out completely with a bleaching of 100 s with blue light. Pulse annealing and impact of bleaching on glow curve suggested the 510°C TL peak (heating rate 2°C/s) to be responsible for the post-500°C pre-heat OSL. This signal would be referred to as deep trap OSL (DT-OSL), emission arising from a trap deeper than 325°C TL trap, in this paper. DT-OSL simply implies that the charge responsible for emission originates from the deep trap and not the mechanism involved in OSL process. This is necessary to differentiate from the conventional OSL (325°C TL Peak). The dosimetric properties of DT-OSL were found to be good in terms of linearity and upper limit of dose estimation. The signal also satisfied various tests needed for reliable use dose estimation.
. Experimental details
The luminescence measurements were carried out on automatic Risø TL/OSL, TL-DA-15 system having a blue light-emitting diodes (λ=470 ± 30nm ) stimulation source with power level set at the 90% of the maximum stimulation intensity for the blue LEDs (40 mW/cm2) (Bøtter-Jensen et al., 1999) It can accommodate 48 samples and has an in-build 90Sr/90Y β-source.. The detection filter used in all observations was a Hoya U-340 (Ip ~ 340 nm, FWHM ~ 80 nm). Irradiation of the samples was carried out using a 90Sr/90Y β-source housed in the system. Also, the bleaching of the specimen was carried out with the stimulation light of the system.
The DT-OSL was measured in case of a Greek geological sample, local ceramic sample and Polish sedimentary sample. Thereafter, the detailed observations were carried out on fired geological Greek sample, Koupa, which acted as a representative sample. It is a milky sedimentary quartz sample collected from the Northern Greece. Apart from utilizing this sample in our recent work (Koul et al., 2014) this sample has also been used by Polymeris et al., (2009) in their earlier work. Grains of dimensions between 90 and 150 μm were obtained from each natural crystal quartz sample after smashing in an agate mortal and wet sieving. These grains were treated with HCI (10%), H2O2 (10%), HF (40%) and a final treatment with HCI (10%) in order to obtain a clean quartz extract. Accordingly, the samples studied were stimulated with IR at room temperature and no significant luminescence was observed. This assured the absence of feldspar in the samples studied in this work (Galloway, 1994).
The samples were fired at 800°C in air in a resistance furnace for one hour and then allowed to cool down in the furnace. Thereafter, all the thermal treatments reported in this work were carried out on the heater of the Risø system. Discs were loaded on alternate slots to reduce the inter-disc interference (Bray et al., 2002). A heating rate of 2°C/s was preferred in TL measurements so as to reduce the temperature lag between the heater plate and the samples. All TL measurements were carried out using a nitrogen atmosphere. The OSL signal in this study was represented by the sum of counts in the initial 5 channels minus the sum of counts in the final 5 channels, with 0.5 s channel width of the recorded shine down curve. All OSL measurements were recorded at a stimulation temperature of 125°C. As indicated above, in this paper the signals corresponding to deep trap will be referred to as deep trap OSL (DT-OSL).
. Nature of the post 500°C blue OSL
To observe the nature of post 500°C OSL or deep trap OSL (DT-OSL), three protocols were devised (i) recording OSL after 500°C pre-heat, (ii) bleaching with blue light of Risø system for 100 s and recording OSL measurement and (iii) recording OSL after bleaching and 500°C pre-heating. The first protocol employed was similar to the one reported in literature (Polymeris et al., 2015; Kitis et al., 2010). These protocols were devised to observe (i) the nature of signal emanating from deep trap, (ii) whether this emission is bleachable and (iii) whether it takes place due to heat treatment only, involving thermally charge transfer mechanism, respectively. The OSL measurements were carried with blue light after an irradiation dose of 200 Gy and pre-heating at 500°C.
All the four samples studied here behaved in a similar way with the above-mentioned protocols as shown in Figs. 1a, 1b, 1c and 1d. A significant signal was observed with pre-heat at 500°C which implied presence of blue OSL originating from deep trap/s as shown in Fig. 1. The absence of OSL recorded after bleaching indicated that the signal emanating from deep trap is bleachable. The last protocol involved bleaching, pre-heating at 500°C and then recording the OSL did not yield any OSL signal, as shown in Fig. 1. This observation suggested that the DT-OSL did not result from thermal charge transfer mechanism, induced with a heat treatment at 500°C in the measurement procedure. Combing the results of the three protocols, it looks obvious that the post 500°C blue stimulated OSL is produced by deeper trap/s and it is easily bleachable.
Fig. 1
OSL decay curves of fired (a) Greek sample, (b) local brick sample (c) Polish sample and (d) Thar dessert samples after 500°C pre-heating (curve ‘A’), bleaching with blue light of RISO system for 100 s (curve ‘B’) and bleaching and 500°C pre-heating (curve ‘C’). The OSL was recorded at a stimulation temperature of 125°C after administering a dose of 200 Gy to the sample. Fresh disc was used for each protocol.
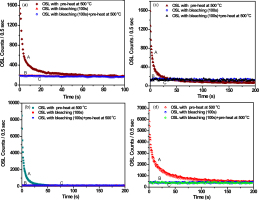
To compare various features of the 510°C TL trap OSL (DT-OSL) with the 325°C TL trap OSL (fast OSL) a protocol was devised which allowed observation of the signals with pre-heating at, both, 260 and 500°C. The Greek sample, Koupa, was irradiated with a dose of 200 Gy, pre-heated at 260°C for 10 s and then stimulated for 200 s at 125°C to record the fast OSL. Thereafter, in order to record the DT-OSL the aliquot was subjected to the same treatment as mentioned above except for the pre-heat, a pre-heat at 500°C instead of 260°C was incorporated. A test dose OSL for sensitivity correction of the two emissions was also recorded using a test dose of 1.5 Gy and cut heat of 160°C subsequent to the OSL measurements.
The OSL decay curves recorded with pre-heats at 260°C and 500°C are shown in Fig. 2; (a) sensitization corrected and (b) corrected signal normalized with respective initial data point. The last plot allowed better comparison of the two emissions. As expected, the relative magnitude of DT-OSL can be seen in Fig. 2b. The DT-OSL signal was more prominent without the application of sensitization correction. This is expected, as the heating at 500°C would induce a larger sensitization of the signal compared to the one observed with heating at 260°C. As is clear from the Fig. 2b, the nature and composition of the two emissions look different. It becomes very clear by plotting the initial 10 data points of two signals as shown in the inset of the Fig. 2b. Comparing the two signals, especially the first ten data points, the DT-OSL does not decay as fast as the fast component, OSL originating from the 325°C TL trap. The DT-OSL does show slower decay behaviour and contained larger slow component as compared to the fast OSL. But, the decay does not seem to be that of the slow component, as reported in the literature.
Fig. 2
OSL shine down curves recorded with pre-heating temperatures of 260°C and 500°C (a) sensitization corrected and (b) the corrected curve normalized with the respective initial data point. The OSL in these plots represent fast OSL (OSL measured with pre-heat at 260°C) and DT-OSL (OSL measured with pre-heat at 500°C). In order to understand the decaying nature of DT-OSL, its initial 10 data points are compared with that of fast OSL in the inset of (b). The shine down curves were corrected for sensitization with respective test dose OSL. These curves were recorded after administering a dose of 200 and test dose of 1.5 Gy. All OSL measurements were done at a stimulation temperature of 125°C.
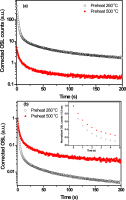
To know more about the nature of the DT-OSL a component analysis of the two signals was done using computerized curve deconvolution analysis (CCDA) (Afouxenidis et al., 2012). The results of the two decay curves recorded with two pre-heating temperatures are shown in Fig. 3; (a) 260 and (b) 500°C. Comparing the two plots it becomes clear that the DT-OSL contains a prominent medium component with decay constant (2.64 s). As expected the OSL measures with 260°C (Fig. 3a) contains a prominent fast component with decay constant (0.957 s), which is missing in case of the signal recorded with pre-heat at 500°C.
. Nature of the DT-OSL trap
In order to identify the trap responsible for DT-OSL phenomenon it was found necessary to observe the (i) nature of the TL glow curve, (ii) impact of bleaching on the TL glow curve and (iii) the pulse annealing behaviour of the OSL signal. The details of these measurements are described as under.
Nature of the TL glow curve
Glow curve gives information about the traps in terms of their number and trap depth. OSL emission has to originate from one or more than one traps corresponding to the glow peaks of the glow curve. TL glow curve of the Koupa sample, as shown in Fig. 4a, was recorded up to temperature of 625°C, with a heating rate 2°C/s, after administration of a dose of 200 Gy. The higher upper recording temperature was chosen to look for glow peaks which correspond to higher temperatures or deeper traps. In addition to all conventional glow peaks reported for quartz, the glow curve also contained a well-defined glow peak at around 510°C. The inset, showing linear log plot of the data, in Fig. 4a presents the glow curve in a better way. In order to see the nature of the 510°C TL peak the glow curve was recorded after thermally cleaning it. This was done by heating the sample up to 475°C and then recording the TL glow curve. The thermally cleaned glow curve, shown in Fig. 4b, can be seen to have a well-defined glow peak at around 510°C, the same temperature as depicted in Fig. 4a. The nature of the glow curve inferred that the DT-OSL might originate from 510°C TL trap.
Fig. 4
(a) TL glow curve of the Koupa, Greek, sample recorded after administration of a dose of 200 Gy and heating up to a temperature of 625°C with a heating rates 2°C/s. The inset in the Fig. 2a shows the high temperatures peaks in a better way by plotting the data in linearlog scale. (b) to have a better feel of the 5l0°C TL glow peak, it was recorded after thermal cleaning of the glow curve by heating the sample up to 475°C.
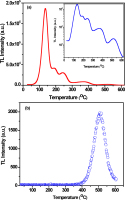
Impact of bleaching on the glow curve
In order to understand the nature of trap responsible for DT-OSL the impact of bleaching on TL glow curve was undertaken. The sample was irradiated with a dose of 200 Gy and then heated to a temperature of 475°C to remove charge from all traps except for the deeper ones, the traps of interest here. Thereafter, the glow curve was recorded up to 575°C at a heating rate of 2°C/s. This curve would be referred to as TL glow curve without bleaching. A test dose 110°C TL was also recorded with a dose of 1.5 Gy for sensitization correction of the glow curve. Since the sample is pre-heated to high temperature of 475°C, before recording of the glow curve, it is expected to get sensitized. Another TL glow curve was measured in an identical way as mentioned above but with a bleaching step of 100 s with blue light of Risø system, at a stimulation temperature of 125°C, before recording the glow curve. This curve would be referred to as glow curve with bleaching and it was, again, corrected for the sensitization the same way as mentioned above in case of TL glow curve measured without bleaching.
Fig. 5 depicts the TL glow curves recorded without prior bleaching and bleaching of 100 s. The comparison of glow curves with and without bleaching identifies the trap or portion of the glow peak which is responsible for the observed OSL. An appreciable depletion in the 510°C TL peak with bleaching is observed, which inferred that this peak is bleachable and, hence, could be responsible for the observed DT-OSL. One can notice from this figure that it is the falling part of the glow peak, higher temperature region, which seems to participate in the production of the OSL signal. It is important to mention here that the thermal quenching encountered at such high temperatures, as administered here in recording the TL glow curve, would be very large (Wintle, 1975). Therefore, the difference between the two curves would be much large than what is visible from the plot once the quenching correction is carried out.
Fig. 5
(a) Sensitization corrected 510°C TL glow peak of Greek sample with and without bleaching treatment. Prior to the TL measurement the sample was heated up to 475°C to remove the traps lower than this glow peak. Heating in both cases, TL measurement and preheating, was done with heating rate of 2°C/s. The bleaching was done for 100 s using the blue light of the Riso system at a stimulation temperature of 125°C. The glow curves were measured with a radiation dose of200 Gy and sensitization corrected with a test dose OSL of 1.5 Gy.
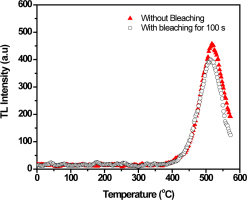
Pulse annealing of OSL emission
This observation involves observing the behaviour of OSL decay curves with different increasing pre-heating temperatures and, thereby, determines the thermal stability of the trap responsible for OSL emission. The observations reported above, suggested that the 510°C TL glow peak could be responsible for DT-OSL. So, accordingly the pre-heating temperature window for the OSL measurements, DT-OSL emissions, was set around this temperature, 450 to 625°C, in steps of 25°C. The protocol devised for this measurement is listed in Table 1. It involved (i) administrating a radiation dose of 100 Gy, (ii) pre-heating at different temperatures starting with 450°C, (iii) recording OSL for 100 s at a stimulation temperature of 125°C, (iv) administrating a test dose of 1.5 Gy and (iv) recording, again, OSL for 100 s at a stimulation temperature of 125°C. The test dose signal measured in step (iv) was used to undertake the sensitization correction of the OSL emission. It is simply done by dividing the signal with the test dose signal. This protocol was repeated with different pre-heating temperatures, as mentioned above.
Table 1
Protocol to record the thermal pulse annealing of deep trap.
The sensitization corrected OSL decay curves recorded with various pre-heating temperatures are depicted in Fig. 6a, the inset represents the un-corrected curves. This figure reveals the existence of an appreciable signal with all pre-heating temperatures, which includes the highest pre-heat at 625°C employed here. The TL glow curves recorded during different pre-heating temperatures are depicted in Fig. 6b. These curves give some insight about the glow peak or trap which can participate in luminescence mechanism with various pre-heating temperatures. This figure clearly demonstrates that the OSL recorded with pre-heating temperatures greater than 450°C cannot be attributed to glow peaks occurring at temperatures lower than 510°C TL peak, as all these peaks get annealed out completely with this pre-heat treatment.
Fig. 6
(a) Plot of sensitization corrected OSL shine down curves as a function of pre-heating temperatures (the inset depicting the uncorrected curves), (b) TL glow curves recorded during pre-heating of 475, 525, 575 and 625°C, applied prior to OSL measurement and (c) pulse annealing curves; plots of sensitization corrected fast OSL signal as a function of pre-heating temperatures, the inset shows a comparison of the sensitization corrected and un-corrected pulse annealing curves. The signal was represented by the sum of counts in the initial 5 channels (2.5 s) minus the sum of counts in the final 5 s of the shine down curve. The sensitization correction was done with a test dose OSL signal. A radiation dose of 100 Gy and a test dose of 1.5 Gy were employed in this measurement. The TL glow curves were recorded with a heating rate of 2°C/s and OSL was recorded at a stimulation temperature of 125°C.
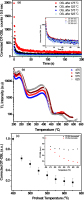
The depletion in the sensitization corrected OSL signal as a function of pre-heating temperature, i.e. pulse annealing curve, is shown in Fig. 6c. The inset of this figure depicts the pulse annealing curves with and without sensitization correction. The erosion of the charge from the trap with increase in the pre-heating temperature would result in the depletion of the signal. As can be seen from the inset of Fig. 6c which has not been un-corrected curve does not show the kind of depletion with increasing pre-heating temperature as is the case with sensitization corrected curves. The reason for this discrepancy is the increase in the luminescence sensitization with increasing pre-heating temperatures, which dilutes the impact of thermal erosion of charge from trap. It must be mentioned here that the magnitude of temperatures employed here would induce significant sensitization, mainly pre-dose sensitization (Zimmerman, 1971; Koul and Chougaonkar, 2007). The corrected thermal pulse annealing curve show appreciable reduction with pre-heating in the temperature region of 450–550°C. So, this measurement correlated with the earlier observation about 510°C TL peak being the trap responsible for production of DT-OSL. The pulse annealing curve depicts that it pre-dominantly emanates from one trap, the 510°C TL trap as depicted in Fig. 6c.
. Bleachability of the DT-OSL trap
The nature of bleaching of the trap responsible for the DT-OSL was observed by measuring the impact of different optical stimulation time periods on the signal. The sample was subjected to a radiation dose of 150 Gy and heated at 500°C, to ensure the removal of charges from traps except for the deep traps. It was then bleached for various time periods starting with 0 s (no bleaching) using the blue light of the Risø system at 125°C. Subsequent to the bleaching, the specimen was stimulated for 200s, again with blue light, at 125°C to record the DT-OSL emission. Finally, the test dose OSL was measured after the administration of a test dose of 5 Gy and cut heat of 160°C. This signal was used to undertake the sensitization correction of the DT-OSL emission. This sequence of measurement was recorded with other bleaching time periods of 0.5, 2, 5, 20, 50 and 100 s.
The sensitization corrected DT-OSL signals observed after various bleaching time durations are depicted in Fig. 7. The correction, as stated above, has been done with the test dose OSL emission. The data in the figure has been divided by the first data point in order to have better feel of the curve. The magnitude of DT-OSL can be seen decaying sharply within first few seconds and reaching almost negligible value after a bleaching of 100 s. This measurement corroborates with the earlier finding, described in Section 3, that the trap responsible for DT-OSL trap is an easily bleachable trap.
Fig. 7
Impact of bleaching on DT-OSL signal with bleaching time periods of 0, 2, 10, 20, 50 and 100 s using blue light of Riso system. The sample was irradiated with a dose of 150 Gy and the bleached at a stimulation temperature of 125°C. The signal was represented by the sum of counts in the initial 5 channels (2.5 s) minus the sum of counts in the final 5 s of the shine down curve. The signal was corrected with a test dose OSL. A dose of 150 Gy was administered to the sample prior to each optical stimulation and a test dose of 5 Gy was used for sensitization correction.
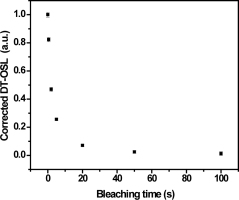
. Dosimetric properties of DT-OSL
The feasibility of any luminescence for dose estimation using depends on its (i) nature of growth with radiation dose, i.e. growth curve, (ii) reproducibility and (iii) degree of reliability in dose estimation (Murray and Wintle, 2000). Accordingly, these measurements were carried out to look into the utilization of DT-OSL for dose estimation.
Nature of growth curve
The protocol employed for recording the growth curve of DT-OSL involved (i) administration of dose, (ii) heating at 500°C, (iii) recording of OSL at a stimulation temperature of 125°C (Lx), (iv) administrating a test dose of 1.5 Gy and (v) recording of OSL at a stimulation temperature of 125°C and a cut heat of 160°C (Tx). The dose values of 0.006, 0.05, 0.3, 0.6, 1, 1.5, 2.5 and 10 kGy and a test dose of 1.5 Gy were employed in this study. To compare the growth curve of this emission from deep trap, DT-OSL, with the 325°C TL trap OSL, fast OSL, the growth curve of the latter was also measured in a similar way as described above but with dose values of 6, 30, 60, 120, 300 and 600 Gy and pre-heat at 260°C. Since the fast OSL typically saturates at around 100 Gy the growth curve was measured up to 600 Gy, only (Aitken, 1998).
The sensitization corrected growth curves (Lx/Tx versus dose) corresponding to DT-OSL and fast OSL emissions with administered dose values up to 1000 and 600 Gy, respectively, are depicted in Fig. 8a. For a better comparison, the data in this plot was normalized in such a way that the data points of the two curves corresponding to 600 Gy, the highest dose used here to record the fast OSL, matched with each other. The growth curves in this plot can be seen to have quite different patterns, while as the fast OSL grows in a non-linear way right from the lowest administered dose, the DT-OSL demonstrates a good degree of linearity till the last data point corresponding to an administered dose of 1000 Gy, as shown in Fig. 8a. The growth curve of the DT-OSL signal recorded with all the administered doses can be to increase continuously till the highest administered dose of 10 kGy, as depicted in Fig. 8b. It has two growth patterns (i) linear till a dose of 1kGy and (ii) non-linear beyond this value of dose (Fig. 8b). The superlinearity index g(D) has evaluated to be equal to 0.97. The DT-OSL growth curve demonstrated its large dynamic range so far dose measurement is concerned. The main interesting feature of this signal is that it can be useful in the upper dose bracket, pushing it into kGy region. As reported in literature and observed here also in Fig. 8a, this is not possible with the fast OSL. This signal has been observed to saturate typically ~ 100 Gy (Aitken, 1998).
Fig. 8
The plot of sensitization corrected signals corresponding to (a) deep trap OSL (DT-OSL) and fast OSL with dose administered up to a dose of 1000 and 600 Gy respectively and (b) only DT-OSL up to highest administered dose value of 10 kGy, the inset in this figure shows plot of the data till a dose of 1 kGy. Since the fast OSL saturated much early than the DT-OSL, so, its growth curve was measured up to 600 Gy only. The data of the two curves in (a) was normalized in such a way that the data points of the two curves corresponding to 600 Gy matched with each other. The protocol involved pre-heats of 260 and 500°C for measurements of fast OSL and DT-OSL respectively and a cut heat of 160°C. The data in DT-OSL could be fitted with a linear fit till a dose of 1 kGy. and latter beyond it till the last administered dose of 2.5 kGy, as shown in (b). The signal was represented by the sum of counts in the initial 5 channels (2.5 s) minus the sum of counts in the final 5 s of the shine down curve. These curves were measured at a stimulation temperature of 125°C.
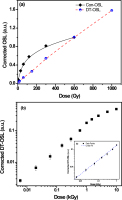
Reproducibility of the signal
Single aliquot regenerative (SAR) protocol has been found to be reliable and efficient in dose estimation using OSL signal (Wintle and Murray, 2006). Good reproducibility of the signal and absence of the thermally transferred charge (termed as recuperation as per the convention of the protocol) determine the reliability of SAR technique. The reproducibility of DT-OSL was undertaken by observing sensitization corrected DT-OSL signal with (i) four identical dose values, (ii) no dose or zero dose and (iii) same dose as administered above once more. This procedure used here is identical to the one employed in SAR, but instead of different regenerative doses an identical dose was employed here. A radiation dose of 100 Gy and test dose of 3 Gy were used in this observation. The measurements were carried out done with a pre-heat and cut-heat of 500 and 160°C respectively. As can be seen from the Fig. 9, a good reproducibility of the signal was observed for all the measurements which, also, include the measurement after the recording of the signal with zero dose value.
Fig. 9
The reproducibility of DT-OSL was undertaken by observing its four repeated sensitization corrected measurements with the same dose value of 100 Gy and then repeating it once more with the same dose, but, after a measurement with administration of a zero dose, i.e. no dose, (run number 5). A test dose of 3 Gy was used in this observation. The measurement was done with a pre-heat and cut-heat of 500 and 160°C respectively. The signal was represented by the sum of counts in the initial 5 channels (2.5 s) minus the sum of counts in the final 5 s of the shine down curve. These curves were measured at a stimulation temperature of 125°C.
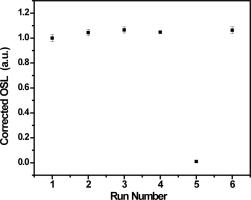
The recuperation term used in SAR protocol, essentially consists of the thermal transfer which might happen during different heating steps of the protocol. The magnitude of this signal is evaluated by measuring the OSL signal with no dose, i.e. zero dose, administered to the sample after various OSL measurements with various regenerative doses. In this observation, no significant recuperation can be seen to take place, as the signal with zero administered dose was observed to be negligible (run number 5 in Fig. 9). This observation, as mentioned above, was made after four OSL measurements, recorded with identical dose of 100 Gy. A recuperation of 0.9% was estimated, which is well within the acceptable limits in case of SAR for dose reliable dose estimation. These observations suggest that SAR could be used satisfactorily for equivalent dose estimation using the DT-OSL emission. Of course, the protocol has to be modified a bit with respect to pre-heating temperature; instead of 260°C for 10 s used in case of fast OSL a pre-heat of 500°C, without holding it, has to be incorporated.
Reliability of dose estimation
Though the DT-OSL has been found above to qualify for dose estimation using SAR, it is the dose recovery test which guarantees the applicability of this protocol for dose estimation. Accordingly, the dose recovery of a fired geological sample utilizing the DT-OSL emission was undertaken. An identical dose of 200 Gy, the dose to be recovered, was administered to three aliquots. The regenerative doses used in the protocol were 100, 300 and 400Gy. A test dose of 1.5 Gy, pre-heat of 500°C (without holding it) and cut heat of 160°C was incorporated in the measurements. The test use signal is used for sensitization correction of the signals.
The equivalent dose estimation using SAR was seen to yield similar results in case of all the three discs employed here. These recovered dose values were 208.06, 204.06 and 206.06 Gy. The average of these numbers is 206.1 ± 1.2 Gy (n=3), which is very close to the administered dose of 200 Gy, giving a dose recovery ratio of 1.030 ± 0.006. This measurement, again, was seen to reproduce the above-mentioned results, i.e. good reproducibility (1.07 ± 0.04) and negligible recuperation (0.9 ± 0.07%). Utilization of high preheating temperatures, as is the case here, completes the sensitization in the first run itself and it might not vary much thereafter. This is reflected in Fig. 10, the luminescence did not show any appreciable sensitization with different runs of the SAR protocol. However, desensitization observed in the last couple of runs seems to occur due to dose quenching as the sample experienced large accumulated dose during SAR protocol. Considering all these results, the DT-OSL seems to be a workable signal for reliable dose estimation. It is important to mention here No clean out stimulation, optical or heating, was required at the end of each SAR cycle in case of DT-OSL. This will be discussed in the next section of the paper.
Fig. 10
The variation in luminescence sensitization during different runs of the SAR. The data represents the average values of the three discs which were administered an identical dose of 200 Gy, i.e. the dose to be recovered. The regenerative doses used in the protocol were 100, 300 and 400 Gy. A test dose of 1.5 Gy and cut heat of 160°C was incorporated in the measurement. The protocol was modified in terms of pre-heating temperature, a heating at 500°C, without holding it for anytime.
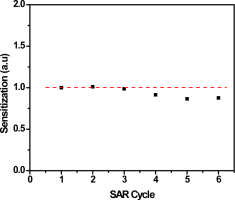
Dating with DT-OSL
Based on the nature of the DT-OSL (i) bleachability, (ii) large dynamic range of the growth curve and (iii) reliability for dose estimation, it will be useful for dating of fired quartz samples. Since the pre-heat of 500°C is incorporated in the measurement of the signal the sensitization during the regenerative protocol would not be appreciable as is the case with conventional OSL. Also based on the dose response curve, as shown in Fig. 8, the DT-OSL will allow to date much older sample than possible with conventional OSL signal. Also since the signal is easily bleachable, no clean-out stimulation was required at the end of each SAR cycle. The proposed protocol for paleo-dose estimation required for dating, as listed below, is just the modified SAR protocol, the pre-heat being 500 instead of 260°C.
Pre-heat the sample with natural dose at 500°C for 10 s
Stimulate for 100 s at 125°C to record the OSL signal (DT-OSLN)
Administer a test dose
Heat at 160°C for 10 s
Stimulate for 100 s at 125°C to record the test dose OSL (OSLTD)
Stimulate at 125°C for 200 s to remove any residual signal
Repeat steps 1) to 7) with different three artificially administered dose values at step 1)
. Discussion and conclusion
The nature of optically stimulated luminescence signal of quartz recorded with high pre-heating temperatures (> 400°C) has been studied in detail by many scientific workers (Jain et al., 2003; Singarayer and Bailey, 2003; Bailey, 2000). The contribution of the fast and medium components to the OSL emission was found to be negligible with such pre-heating temperatures. It is the slow component, only, which has been reported to survive with such pre-heating temperatures. This emission is characterized by a slowly decaying emission; the complete bleaching of this signal could take as much as two weeks with exposure to sunlight. However, the nature of signal reported with high pre-heating temperatures in this study yielded an appreciable medium component apart from the conventionally observed slow component in all the four fired samples studied here. The decay was seen to be much faster than what is expected from a slow OSL, as is shown in Fig. 1. This was validated by the results obtained with the component analysis, which showed the presence of a dominant medium component in the observed OSL, decaying much faster than the slow component (Fig. 3). This emission was, also, seen to get bleached out almost completely within 100 s of stimulation with blue light, which is impossible in case of slow component of OSL emission. So, the signal reported here being un-conventional, medium but recorded with high pre-heating temperature, and emanating from deep trap has been termed as deep trap OSL (DT-OSL). The nature of glow curve indicated that the deep trap which could contribute to DT-OSL to be the 510°C TL peak, heating rate of 2°C/s. This signal was found to be very weak as compared to fast OSL, i.e. signal measured with pre-heat of 260°C, their intensities being in the ratio of 1 : 0.06.
The thermal pulse annealing characteristics of the luminescence with pre-heat showed an appreciable decrease in the DT-OSL between heating temperatures of 450 and 550°C (Fig. 6c). This measurement suggested the trap corresponding to 510°C TL peak to be mainly responsible for the DT-OSL. The impact of bleaching on 510°C glow peak corroborated the above mentioned finding about this TL peak being responsible for the DT-OSL. The comparison of TL glow peaks, with and without bleaching, demonstrated the participation of this peak in the luminescence process (Fig. 5). One can see from this figure that it is the falling part of the peak which contributes to the luminescence phenomenon (Fig. 5).
The nature of growth curve of DT-OSL, undertaken to look into its feasibility for equivalent dose estimation, was seen to increase up to the largest dose applied in this study, 10 kGy as shown in Fig. 8a. So, the dose response of this signal is extremely high as compared to that of the fast OSL, which generally saturates at around 100 Gy (Murray and Wintle, 2000; Koul and Chougaonkar, 2007). In this study, also, the fast OSL emission was seen to saturate much early than the DT-OSL (Fig. 8b). Moreover, the growth curve of DT-OSL contained a large initial linear part up to a dose of 1000 Gy, which, again, is not true of the fast OSL. The latter generally follows a non-linear growth with all range of doses. These observations implied that incorporating this signal could be useful for dosimetric applications involving higher dose measurements, in the kGy region of fired quartz samples.
The DT-OSL signal was observed to fulfill the necessary conditions required for the reliable dose estimation. Both, reproducibly and dose recovery was observed to be quite good. There was almost negligible recuperation, i.e. transfer of charge among different traps, while executing the SAR protocol for dose evaluation. It is worthwhile to mention here that no clean-out stimulation was required at the end of each SAR cycle with this signal, as has been the case with TT-OSL and post-blue violet stimulated luminescence signals (Adamiec et al., 2010; Hernandez and Mercier, 2015). The reason for this being that the DT-OSL, the signal employed in this work, is easily bleached out. Another good feature about this signal is that since the protocol involves a high pre-heating temperature the sensitization gets almost completed in the first run of SAR, itself. Thereafter there is not much change in the sensitization during subsequent runs (Fig. 10).
Thermal treatment, firing, has been observed to affect luminescence properties of quartz (Sankaran et al., 1983). Firing at higher temperature has been observed to destroy the radiation sensitive traps and create thermally sensitive traps and, on the contrary, the growth defects disappear after prolonged irradiation. This sort of transformation is primarily activated by the kinetics of the monovalent alkali ions. They prefer to flip-flop in the lattice during heating and irradiation treatment, so as to choose their preferable partners (Jani et al., 1983a, 1983b).
In a recent study involving the impact of firing on the nature of CW-OSL of quartz it was observed that the composition of the OSL signal changed significantly (Koul et al., 2016). While as the signal in case of natural sample was dominated by the slow component, it has been the components other than the slow component which dominated the signal in case of the fired sample. Added to this feature the large sensitization encountered by sample with firing, the DT-OSL would be dominant in case of fired specimen as compared to the un-fired ones. So, the signal even if present in un-fired specimen might be too small in magnitude to get noticed in presence of the large slow component. This might be the reason why this emission has not been reported in case of quartz derived from unfired samples, sediments. Considering the results of this study, the DT-OSL signal observed for fired quartz sample might be useful in applications involving higher dose evaluation, like dating, food irradiation, sludge treatment etc.