. Introduction
The Roman amphitheatre in Emerita Augusta or present day Mérida, was sampled in July 2000 at an early stage of the development of radiocarbon dating methods for lime mortars using partial dissolution and AMS. The goal at this stage was to test the suitability of different samples for radiocarbon dating. The city had been founded by Emperor Augustus in 25 BC for retired soldiers (Ortiz et al., 2014), but the consensus was that the amphitheatre would be Flavian, i.e. late 1st c. AD (Mateo Cruz, director of the Museum, oral communication at the sampling site). The samples collected were different in many ways compared with what we had encountered earlier including Roman samples from Rome and Torre de Palma, Eastern Portugal (Ringbom et al., 2006, 2011; Lindroos et al., 2011; Langley et al., 2011). The samples were remarkably hard, but they did not classify as hydraulic based on the chemical composition according to a classification used by Van Strydonck et al., (1986) and because the carbon yield was similar to that of many lime mortars, between 5.6% and 8.0%. Apparently, however, the hardness seems to be linked to poor permeability for carbon dioxide (CO2) leading to delayed hardening of the mortars, as all four samples had readily soluble carbonate that yielded unreasonably young ages. One of the samples (Mérida 003) was dated by sequential dissolution (Lindroos et al., 2019a) in many CO2 fractions: The first time in only two CO2 fractions within 13 min and the second time with total dissolution in 13 hours and six consecutive CO2 fractions. It turned out that after about 10 min of dissolution with 85% phosphoric acid (H3PO4) the effervescing CO2 started yielding 1st c. AD ages quite consistently. The result from this one sample was published (Hale et al., 2003; Ringbom et al., 2006; Lindroos et al. 2020. According to the interpretation we made, combining the results from CO2 fractions 3, 4 and 5 the age was AD 5-90 with 86.9% probability at 95.4% confidence level or AD 100123 with 8.5% probability (c2-Test: df 2, T=3.2 (5% 6.0)).
A comprehensive description of the Roman mortars in Mérida was made recently (Mota-Lopes et al., 2018) and an Augustan, early AD 1st c. age was claimed. We therefore decided to revisit the samples in order to test whether the other samples would also yield 1st c. AD ages later on in the hydrolysis process and if it would be possible to get a better resolution for the chronology. Because there were unspecified carbonates causing young ages early in the dissolution progress, we also tested if it was possible to get rid of some of them by heating the sample before hydrolysis.
. Material and methods
Mérida in SW Spain was the capital of the Roman province Lusitania. It was founded as Emerita Augusta by Emperor Augustus in 25 BC as a retirement place for merited soldiers from the Cantabrian wars (Ortiz et al., 2014). Of the main public buildings, the theatre and amphitheatre are preserved and stand next to each other. They were declared the UNESCO World Heritage site Emerita Augusta in 1993 (UNESCO 2015). The amphitheatre is an oval construction 126.3 by 102.7 m with a central arena of 64.5 by 41.2 m (Capello and Galán 1995). The buildings and the geological building materials are described in detail by Mota-Lopes et al., (2018). In 2000 we took four samples from the amphitheatre (Fig. 1):
Fig 1
Sample positions 1-4 in the amphitheatre. Sampling: Upper left, Mérida 001; Upper right, Mérida 002; Lower left, Mérida 003; Lower right, Mérida 004. See details in the text.
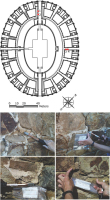
Sample Mérida 001 from the main portal towards the theatre, the south side of an arched passageway, ca 2 m above ground level.
Sample Mérida 002, from the main portal leading to the theatre, arched passage, south wall, ca 1.5 m above ground level.
Sample Mérida 003, from the top floor, passage towards the north east, very thin surface piece, high up on the eastern side of passageway.
Sample Mérida 004, from the top floor, passage towards the north east, surface, high up on the wall.
The samples were inspected visually and with a stereo microscope. From sample 003 we made a thin section for petrographic microscopy. The sample preparation for 14C measurements included:
Crushing with plastic covered pliers to <5 mm pieces and powder.
Sieving in a mechanical sieve vibrator for 20 min into grain-size fractions >500μm, 301-500μm, 151-300μm, 101-150μm, 76-100μm and ≤75μm. Then wet-sieving/washing of the finest fraction to 76-100μm, 46-75μm, 21-45μm and ≤20μm. The washing included spraying the grain-size fractions with de-ionized water in the sieves.
Checking the alkalinity of the sample. In 2000 the procedure included putting about 100 mg of the 301-500μm fraction in a small beaker with the addition of two drops of phenolphthalein solution (2g powder in 100g alcohol) on the sample powder and then filling the beaker up with 10 ml of distilled water. Alkaline samples would turn the water aniline red immediately. When we re-dated the samples in 2018 we also measured the alkalinity with pH strips after one minute and again after 5 min.
Choosing a grain-size fraction for dating and examining it with cathodoluminescence. In this study all the hydrolysed and dated sample aliquots were 46-75μm.
Analysing the chemical composition with ICP-OES at Geological Survey, Finland or at the Institute of Analytical Chemistry, Åbo Akademi University (reference samples Mérida 005-007). 100mg of the 150-300μm grain-size fraction was dissolved in 1M HCl at room temperature for one hour and then diluted to 1/50 and analysed. All elements analysed were well over the quantification limits except for potassium.
Aliquots of the dated 46-75μm fractions were analysed with TGA (ThermoGravimetric Analysis) at the Institute of Inorganic Chemistry, Åbo Akademi University. About 10mg powder was heated gradually from 20-900˚C, 10˚/min in nitrogen atmosphere.
Hydrolysis with 85% H3PO4 at 0˚C from an ice bath. In 2001 the hydrolysis was done at the Aarhus AMS Laboratory. A reactor vessel with a side arm containing the acid was used. When sufficient vacuum was reached in the preparation line, the vessel was tilted, so that the acid flowed onto the sample powder. The hydrolysis was done twice: First to determine the total CO2 yield and the second time to collect two CO2 fractions from the beginning of the acid reaction. The first fraction was collected within 14-104s and the second fraction in 3-14min. Samples Mérida 001 and 003 were re-dated. The former in a 10min first CO2 fraction followed by a 37min second CO2 fraction and the latter in six CO2 fractions spanning from 30s to 13h. Re-dating in 2018 was done using the dedicated preparation line (Ringbom, 2014; Lindroos et al., 2018, 2019a) at Åbo Akademi University, Finland. Smaller CO2 increments could now be collected and the resolution between carbonate phases with different 14C ages and dissolution rates was improved. Since the samples had produced reasonable and consistent ages rather late in the hydrolysis progress the number of late CO2 fractions was increased (see appendix) while some of the early CO2 fractions were left undated. As it had turned out earlier that young carbonates was the major problem and dead carbon contamination only a minor problem, we also tested dating an aliquot that had been pre-heated to 620°C before hydrolysis with the intention to de-carbonate thermally less stable minerals than calcite.
AMS data is reported according to the convention of Stuiver and Polach (1977) and calibrations were done with the program OxCal 4.3 (Bronk-Ramsey (2017), using IntCal 9 data (Reimer et al., 2013). Old Aarhus AMS data come from the EN tandem accelerator and the sample preheating data was performed with the Aarhus HVE 1 MeV accelerator. Zürich measurements were carried out with the ETHZ MICADAS 200 keV accelerator (Synal et al., 2007). δ13C values for old Aarhus data were measured on the GV Instruments Isoprime stable isotope mass spectrometer at the Science Institute in Reykjavik, Iceland on CO2 splits before graphitization. For later Aarhus data, δ13C values were similarly obtained from off-line stable isotope mass spectrometry at the AMS Centre. The Zürich data have δ values measured from graphite in the AMS.
. Results
All samples passed the alkalinity test. pH strips gave values 6-7 and the phenolphthalein test gave a mild reaction, which was only slightly stronger for sample Mérida 002. The samples have a fine-grained aggregate composed of angular quartz and feldspar grains and pieces of mica schist. The angular shape suggests crushing of material rather than use of natural sand. There are only few small voids and some of them have secondary calcite along the walls. Lime lumps are small and scarce, mm-size and they are white to greyish. In CL the calcite binder appears red, indicating a high magnesium content (Marshall, 1988). However, in the 46-75μm grain-size fraction for dating the binder grains are dark. Only a few red grains appear (Fig. 2).
Fig 2
Thin section images. Upper left: 100μm section of sample Mérida 001 in transmitted light. Transparent minerals are mostly quartz. CL images: Upper right, cut and polished surface of a piece from sample Mérida 003 showing distribution between calcite (red) and other binder minerals (black). Mid left, the dated 46-75μm grainsize fraction of sample Mérida 001; Mid right, Mérida 003 ditto. Lower left, Mérida 004 ditto. Images from sample Mérida 002 are omitted because it turned out to be younger and less relevant. Red minerals are calcite and dark red ones have a dolomitic component while more orange ones are purer. Blue and turquoise are quartz and dull green are feldspar. The green dust-like powder in the slice is secondary aragonite from grinding. There is also a void filled with an extremely luminescent mineral in lilac, which we have not tried to identify.
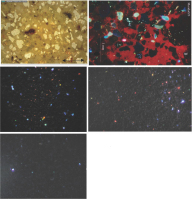
Chemically the soluble material of the samples is unique: i.e. completely different from hydraulic samples in Rome, and also quite different from medieval mortars in Mérida, except for sample Mérida 005 from Bishop Masonas Hospital in Mérida that resembles the amphitheatre samples (Table 1). Very low Al, Fe and Si values and high Ca and Mg values result in a very low hydraulic index, 0.02-0.03 defined as:
Table 1
ICP-OES analyses of the soluble phase of the samples when dissolved for 1h in 1M HCl at room T. For comparison, 3 samples from the Medieval Bishop Masonas Hospital (Mérida 005, 006 and 007) and 3 samples from the Colosseum are included. Major elements are converted to oxides.
A ratio >0.5 is considered to indicate hydraulicity. The chemical analyses verifies the high Mg content deduced from CL color of the binder.
When considering hydraulic properties as a ratio between carbonate CO2 and the amount of OH- bound to minerals as crystal water the situation is completely different. In TGA we consider the loss on ignition (LOI) between 550˚-800˚ as CO2 from carbonate and LOI 250˚-550˚ as crystal water from hydraulic minerals and a ratio CO2/OH- (wt) <10 as hydraulic (Modified from Bakolas et al., 1998; Moropoulou et al., 2005). In that case, samples Mérida 001 and 002 are hydraulic but less so than samples Mérida 003 and 004 (Table 2). The CO2 yield when the samples were hydrolysed for dating in 2018 is also listed.
Table 2
Numerical TGA data and the CO2 yield in H3PO4 hydrolysis for aliquots of the same sample material. For TGA about 10mg of the 46-75μm was heated and in the hydrolysis about 50mg was reacted with 85% H3PO4. The acid was taken from an ice bath but it reached room T before the reaction ceased after about 1h.
Fig. 3 presents the actual TGA profiles
Fig 3
TGA profiles of the four samples from Mérida. The profiles are similar, but the most hydraulic samples Mérida 003 and 004 have lower CO2 yields and consequently more residue.
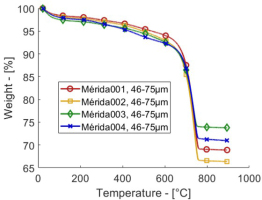
In the appendix there is detailed numerical hydrolysis and AMS data for the samples.
The 14C data is presented below as functions of the dissolution progress variable F defining 14C profiles (F is the ratio between the CO2 produced at a certain time relative the total CO2 yield). Sample Mérida 001 is presented in Fig. 4:
Fig 4
14C profiles from three different sample preparations of sample Mérida 001: Open circles are from the first dating attempt in 2001. Open boxes are from supplementary measurements later the same year and black diamonds from re-dating 2018. The grey bars along the abscissa denote the size of each CO2 fraction relative to the total CO2 yield. In all the following 14C profile plots the size of the CO2 fractions will be presented the same way without specific mention.
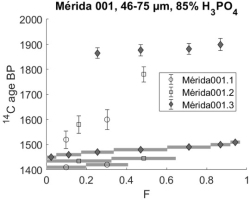
Sample Mérida 002 has the following profiles (Fig. 5).
Fig 5
14C profiles of sample Mérida 002. Open circles present dating in 2001 and black boxes dating in 2018.
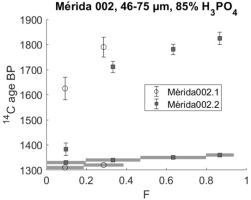
The sample has similar profiles to the others, but the BP ages within the profile (2018) are not overlapping and, even in later fractions, the sample appears about a century younger than the other ones.
Sample Mérida 003 was the subject of four different dating attempts. Fig. 6 shows profiles comparable with those of Mérida 001 and 002. A calibration (AD 25-85; at 58.8% probability and AD 100-120 at 9.4% ditto), based on the boxes 3, 4 and 5 3, 4 and 5 defining a plateau in the profile is presented in Hale et al., (2003) and Ringbom et al., (2006). In 2018 we tested the storage stability of the sample. The 46-75μm sample powder had been in a non-airtight container for 17 years, however, re-dating, did not reveal significant modern carbon absorption, at least not among readily soluble carbonates.
Fig 6
14C profiles of sample Mérida 003. Open circles denote dating in 2001 and open boxes a profile in six CO2 fractions dated later the same year. Black diamonds represent re-dating in 2018. For this sample we used the same powder that had been in a non-airtight container for 17 years. The data point with low F value representing initially effervesced CO2 was measured to ensure that the sample powder had not captured modern CO2 and grown new calcite on the grains.
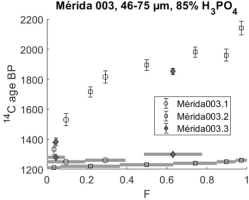
We tested heating the samples before doing the hydrolysis. In our first experiment we applied pre-heating at 620˚C only. Fig. 7 shows the result.
Fig 7
14C profile from sample Mérida 003 after heating the sample to 620˚C. Some of the young carbonates have been lost and the profile now increases more rapidly in the beginning compared with the profile from the non-heated original sample powder (Fig. 6).
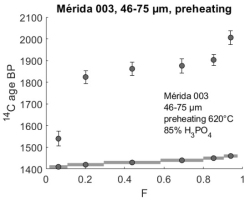
Sample Mérida 004 was also dated both in 2001 and in 2018. Fig. 8 presents the results graphically.
The sample is similar to the others; with readily dissolved young carbonates and ages stabilizing near 1900 BP in later CO2 fractions.
. Discussion
Dating of the Mérida amphitheatre was attempted already in 2001 but the 14C ages were not conclusive and only a small part of the data was published, and in graphical form only. It was clear that the samples were difficult to date because in hydrolysis the initially produced CO2 yielded variable and unreasonably young ages. However, a profile with 14C measurements from six CO2 fractions yielded 1st c.
AD ages for fractions 3, 4 and 5. We therefore also tested the other samples to see whether they would produce similar profiles. It turned out that they did and we now have seven new measurements from rather late CO2 fractions in the hydrolysis and they all yield 14C BP ages in the range 1850-1930 (Fig. 9). Furthermore the CO2 of this age is the main component in the three relevant samples comprising >50% of the carbon inventory. One sample, Mérida 002, seems younger, but without a conclusive age.
Fig 9
14C data from 2018. Data points for each sample are connected with lines for clarity. Samples Mérida 001, 003 and 004 yield similar 14C ages after about 20% dissolution (F>0.2) whereas sample Mérida 002 appears younger in all fractions.
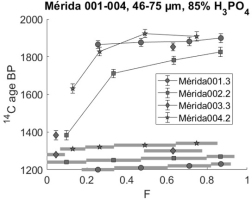
A combined calibration of the five oldest CO2 fractions mentioned was conducted (Fig. 10) and based on these samples, the amphitheatre is Flavian and not Augustan. Any post-Flavian age is not discussed here.
Fig 10
Combined calibration of 5 CO2 fractions with similar ages. The calibration includes the 3 last fractions from sample Mérida 001 and the two last ones from Merida 004 in Fig 9.
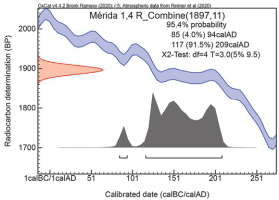
Pre-heating the sample Mérida 003 yields similar results but some of the contamination from young carbonates in early CO2 fractions have disappeared. The role of the magnesium-rich binder is unclear. It probably slows down the hydrolysis and the Mg part should release CO2 earlier than the Ca part when heated (Deer et al., 1992). Heating the sample before hydrolysis is yet an unexplored field, which may have potential in the future especially when dating both the thermally released CO2 and the carbonate residue left for the hydrolysis (Lindroos et al., 2019b).
. Summary
Some mortar samples are difficult to date and require many 14C measurements before a conclusive age can be reached. The situation is especially difficult when the sample has readily soluble carbonate that yields erroneous ages or if the proper binder dissolves very slowly and has a low carbonate content. In the case of the Mérida amphitheatre, there is a relatively slowly dissolving hard binder with a high magnesium concentration and as yet unidentified carbonate minerals, of which at least part of them can be thermally decomposed at lower temperature than the proper binder calcite. The binder should, however, be the main carbonate component and multiple measurements should identify it and reveal its age. Three of the samples point at a Flavian age and none of them at an Augustan age.